Two Pex5 Proteins With Different Cargo Specificity Are Critical for Peroxisome Function in Ustilago maydis
- 1Department of Biology, Philipps-University Marburg, Marburg, Germany
- 2Institute of Metabolism and Systems Research (IMSR), and Centre of Membrane Proteins and Receptors (COMPARE), University of Birmingham, Birmingham, United Kingdom
- 3Center for Synthetic Microbiology, Philipps-University Marburg, Marburg, Germany
Peroxisomes are dynamic multipurpose organelles with a major function in fatty acid oxidation and breakdown of hydrogen peroxide. Many proteins destined for the peroxisomal matrix contain a C-terminal peroxisomal targeting signal type 1 (PTS1), which is recognized by tetratricopeptide repeat (TPR) proteins of the Pex5 family. Various species express at least two different Pex5 proteins, but how this contributes to protein import and organelle function is not fully understood. Here, we analyzed truncated and chimeric variants of two Pex5 proteins, Pex5a and Pex5b, from the fungus Ustilago maydis. Both proteins are required for optimal growth on oleic acid-containing medium. The N-terminal domain (NTD) of Pex5b is critical for import of all investigated peroxisomal matrix proteins including PTS2 proteins and at least one protein without a canonical PTS. In contrast, the NTD of Pex5a is not sufficient for translocation of peroxisomal matrix proteins. In the presence of Pex5b, however, specific cargo can be imported via this domain of Pex5a. The TPR domains of Pex5a and Pex5b differ in their affinity to variations of the PTS1 motif and thus can mediate import of different subsets of matrix proteins. Together, our data reveal that U. maydis employs versatile targeting modules to control peroxisome function. These findings will promote our understanding of peroxisomal protein import also in other biological systems.
Introduction
In eukaryotic cells specific metabolic pathways are often contained inside of organelles such as mitochondria and peroxisomes (Lodish et al., 2000). Peroxisomes have versatile biological roles including detoxification of hydrogen peroxide, degradation of fatty acids and metabolism of amino acids and are essential for human health (Smith and Aitchison, 2013; Wanders, 2014). Peroxisomal matrix proteins are imported into the organelle lumen from the cytosol via an evolutionary conserved set of cytosolic receptors and peroxisomal membrane proteins (Gabaldón, 2010; Kim and Hettema, 2015; Walter and Erdmann, 2019). The majority of known matrix proteins contains one of two conserved targeting signals termed peroxisomal targeting signal type 1 (PTS1) and type 2 (PTS2). PTS1 motifs are located at the C-terminus, originally identified as a tripeptide with the sequence Ser-Lys-Leu (SKL), occurring in many variations of this prototype sequence (Gould et al., 1989; Brocard and Hartig, 2006; Lingner et al., 2011; Nötzel et al., 2016). The amino acid sequence upstream of the C-terminal tripeptide contributes to PTS1 recognition (Brocard and Hartig, 2006). PTS2 motifs are located in the N-terminal part of a protein and are recognized by the receptor protein Pex7 (Braverman et al., 1997; Lazarow, 2006; Kunze et al., 2011; Kunze, 2020). Several proteins lacking canonical targeting signals have been described (van der Klei and Veenhuis, 2006). These either rely on piggy-back import mediated by their interaction with other PTS-containing proteins (Glover et al., 1994; McNew and Goodman, 1994; Islinger et al., 2009; Schueren et al., 2014; Effelsberg et al., 2015; Saryi et al., 2017; Gabay-Maskit et al., 2020) or on direct interaction with Pex5 (Skoneczny and Lazarow, 1998; Klein et al., 2002; Rymer et al., 2018; Kempiński et al., 2020; Rosenthal et al., 2020; Yifrach et al., 2021).
Pex5 recognizes PTS1 motifs via several tetratricopeptide repeats (TPRs) located in its C-terminal domain (CTD) (Brocard et al., 1994; Gatto et al., 2000). Subsequently, the receptor-cargo complex interacts with the peroxisomal membrane proteins Pex13 and Pex14 (Gould et al., 1996; Urquhart et al., 2000; Lill et al., 2020), followed by import of cargo proteins into the peroxisomal lumen without the requirement for ATP turnover (for review see: Miyata and Fujiki, 2005; Kim and Hettema, 2015; Francisco et al., 2017). The interaction with Pex14 is mediated via a conserved di-aromatic amino acid motif located within the unstructured N-terminal domain (NTD) of Pex5 (Saidowsky et al., 2001; Otera et al., 2002; Carvalho et al., 2006; Su et al., 2009). The exact mechanistic functionality of the translocation machinery is still a matter of investigation and so far lacks structural data, but probably has a transient character (Meinecke et al., 2010; Dias et al., 2017; Bürgi et al., 2021). After cargo release, Pex5 proteins are recycled from the peroxisome to the cytosol. Recycling involves ubiquitination, unfolding, energy provided by the AAA-ATPases Pex1 and Pex6, and deubiquitination (Miyata and Fujiki, 2005; Platta et al., 2005; Platta et al., 2007; Gardner et al., 2018; Pedrosa et al., 2018; El Magraoui et al., 2019).
In mammals, two isoforms of Pex5 – Pex5-small and Pex5-large – are generated that derive from alternative splicing (Braverman et al., 1998). The longer isoform contains an additional Pex7 binding domain inside of the NTD. In mammals, another TPR-containing protein with significant homology to Pex5 was identified, which can interact with PTS1 proteins but also with an ion channel (Amery et al., 2001; Santoro et al., 2004, 2011). Many fungi encode two proteins with homology to the PTS1 receptor Pex5 (Kiel et al., 2006; Freitag et al., 2012). In Saccharomyces cerevisae, the Pex5 paralog Pex9 is induced in cells grown in oleic acid-containing medium and controls peroxisomal import of the glyoxylate cycle enzyme malate synthase and additional cargo (Effelsberg et al., 2016; Yifrach et al., 2016; Yifrach et al., 2022). Thus, distinct pathways to target PTS1 proteins to peroxisomes are found in diverse eukaryotes and may be crucial for the regulation of peroxisomal protein import and peroxisome function.
In the phytopathogenic fungus U. maydis, which causes smut disease on corn (Lanver et al., 2017), we have identified two Pex5-paralogs – termed Pex5a and Pex5b (Freitag et al., 2012). Pex5b is the longer paralog and contains a putative binding domain for the PTS2 receptor Pex7 (Figure 1A). Here, we show that the NTDs and the C-terminal TPR-domains of Pex5a and Pex5b each can recognize and import specific cargo. Additionally, we found that the NTD of Pex5b is essential for import of all peroxisomal matrix proteins investigated, and thus acts as a critical component in a dynamic network of receptors that target soluble proteins into peroxisomes.
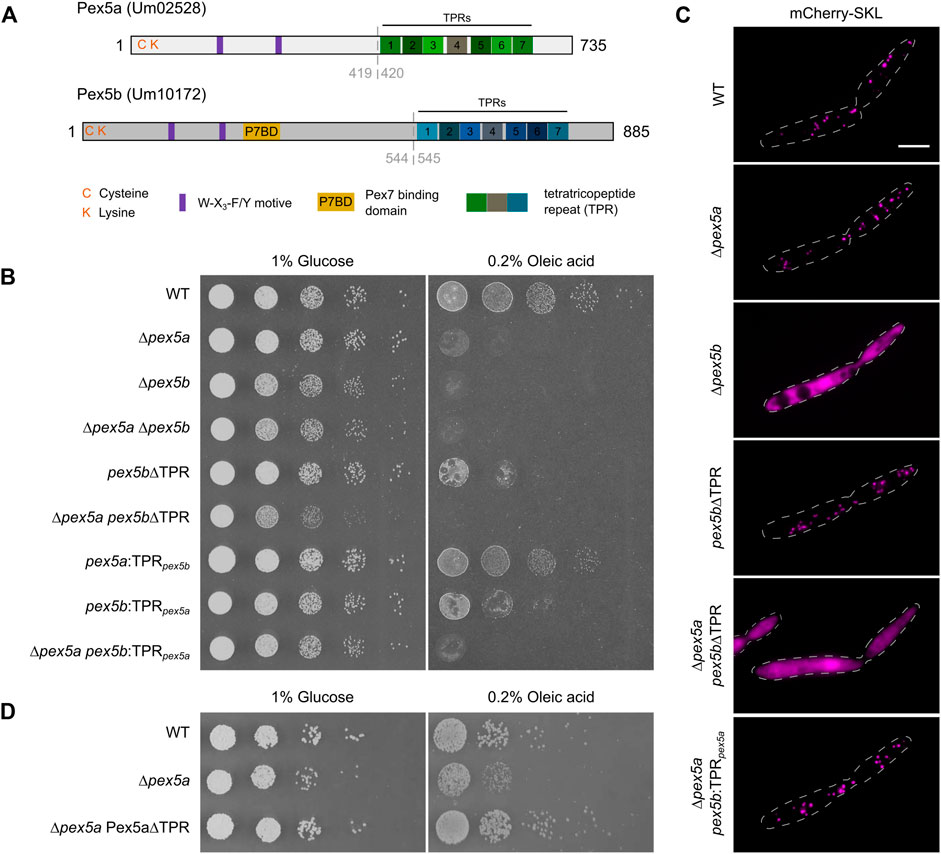
FIGURE 1. Contribution of the N- and C-terminal domains of two Pex5 proteins to peroxisome function. (A) Scheme illustrating the domain organization of Pex5a and Pex5b from U. maydis. C and K refer to a cysteine and a lysine residue possibly involved in ubiquitination. Purple rectangles denote the position of W-X3-F/Y motifs required for docking complex interaction. A putative Pex7 binding domain (P7BD) of Pex5b is shown in yellow. The position of the TPR domains is highlighted with rectangles. Gray dashed lines indicate the position of domain swaps to generate chimeras (see Supplementary Figure S3). (B) Serial tenfold dilutions of indicated strains were spotted on minimal media containing either glucose or oleic acid as sole carbon source. Plates were photographed after 2 days of incubation at 23°C. The assays reveal that both the NTD and the CTD of Pex5a and Pex5b contribute to peroxisome function to a different extent. It is yet unclear why growth of mutants only expressing the NTD of Pex5b is heavily affected on glucose media. (C) Fluorescence microscopic images of indicated strains expressing the peroxisomal marker protein mCherry-SKL. Scale bar represents 5 µm. Notably, the ∆pex5a pex5b:TPRpex5a mutant shows peroxisomal localization of mCherry-SKL but is heavily affected in the growth assays (Figure 1B). (D) Serial tenfold dilutions of wild type cells, ∆pex5a mutants and ∆pex5a mutants overexpressing the NTD of Pex5a were spotted on minimal media containing either glucose or oleic acid as sole carbon source. Plates were photographed after 3 days of incubation at 23°C. Accordingly, Pex5a∆TPR is a functional targeting factor.
Results
Functional Analysis of Pex5a and Pex5b From U. maydis
In a previous study we have described two Pex5-like proteins, Pex5a (Um02528) and Pex5b (Um10172) from U. maydis required for optimal growth of the fungus in different nutrient conditions and for pathogenic development (Figure 1A; Freitag et al., 2012). To discriminate the specific contributions of both proteins for growth on different carbon sources (Freitag et al., 2012; Camões et al., 2015), we tested pex5a and pex5b single and double deletion mutants on media containing either glucose or oleic acid as sole carbon source (Figure 1B). We found that ∆pex5a cells did not exhibit a severe growth defect on glucose plates but on oleic acid-containing medium (Figure 1B). Growth of mutants lacking pex5b or both genes was reduced on glucose-containing medium indicated by smaller colonies on solid medium and lower growth rates in liquid medium (Figure 1B and Supplementary Figure S1). On plates containing oleic acid as sole carbon source proliferation of these mutants was almost abolished (Figure 1B). This indicates that the presence of both Pex5 proteins is required for peroxisome function in U. maydis.
Next, we followed localization of the peroxisomal reporter protein mCherry-SKL in ∆pex5a and ∆pex5b cells (Figure 1C). Peroxisomal localization of mCherry-SKL was observed in ∆pex5a mutants but not in ∆pex5b mutants suggesting that Pex5b is necessary for peroxisomal import of PTS1 proteins in U. maydis. To test whether impaired binding of PTS1 cargo was responsible for the phenotype of ∆pex5b cells we deleted the TPR domains of Pex5b. Although the partial deletion of pex5b affected growth on oleic acid-containing medium (Figure 1B), pex5b∆TPR mutants still were able to import mCherry-SKL into peroxisomes (Figure 1C). Pex5b can therefore import PTS1 proteins into peroxisomes in the absence of Pex5a, while Pex5a-mediated protein import depends on the Pex5b NTD.
To address whether the observed growth phenotype of ∆pex5a cells (Figure 1B) results from different specificities of the Pex5a and Pex5b receptors for distinct subsets of peroxisomal matrix proteins or is caused by the reduced overall amount of Pex5 receptors, we overexpressed Pex5 derivatives. Overexpression of Pex5b rescued the growth phenotype of ∆pex5b cells but did not restore the growth phenotype of ∆pex5a cells (Supplementary Figures S2A,B). This indicates that it is not the reduced dosage of TPR proteins but rather the specificity of the Pex5a receptor, which explains the phenotype of ∆pex5a mutants.
We addressed this hypothesis by construction of strains expressing Pex5a and Pex5b chimeric variants (Supplementary Figure S3). A mutant expressing Pex5 proteins containing only the TPR domain of Pex5a (pex5b:TPRpex5a) exhibited a much stronger growth defect on oleic acid-containing medium compared to a mutant expressing only the TPR domain of Pex5b (pex5a:TPRpex5b), which only showed a slightly reduced colony size (Figure 1B). The TPR domain of Pex5b, therefore, recognizes specific PTS1 proteins required for peroxisome function in these conditions. This is further supported by the finding that, although growth was abolished on oleic acid-containing medium (Figure 1B), mCherry-SKL is localized to peroxisomes in ∆pex5a pex5b:TPRpex5a cells indicating that this chimeric protein is not generally affected in peroxisomal import of PTS1 proteins (Figure 1C).
In addition, our experiments reveal that the absence of the NTD of Pex5a is primarily responsible for the growth defect of the ∆pex5a strain on oleic acid-containing medium (Figure 1B, compare mutant ∆pex5a with pex5a:TPRpex5b). Indeed, overexpression of Pex5a∆TPR was able to rescue the phenotype of a ∆pex5a strain (Figure 1D). Critical peroxisomal matrix proteins are likely to exist, which specifically require the NTD for import. Moreover, the data suggest that Pex5a can act as a targeting factor even in the absence of its TPR domains.
Identification of Pex5a-Specific Cargo
Several proteins from other fungi are known, which are imported into peroxisomes via binding to the NTD of Pex5 although they lack a canonical PTS or the PTS1 has been removed (van der Klei and Veenhuis, 2006). We reasoned that PTS1-containing substrates that require the NTD of Pex5a may as well display specificity for the TPR domain of this cargo receptor. To identify such proteins, we generated a library of GFP proteins with C-terminal dodecameric PTS1 motifs derived from U. maydis enzymes presumably involved in peroxisomal ß-oxidation (Table 1; Figure 2A and Supplementary Figure S4; Brocard and Hartig, 2006; Camões et al., 2015). Constructs were expressed in WT and in ∆pex5a cells (Supplementary Figure S4). GFP fused to PTS1 motifs of the candidate proteins Um01966, Um10665 and Um11001 showed peroxisomal localization in WT cells but accumulated in the cytosol in the absence of Pex5a (Figure 2A and Supplementary Figure S4).
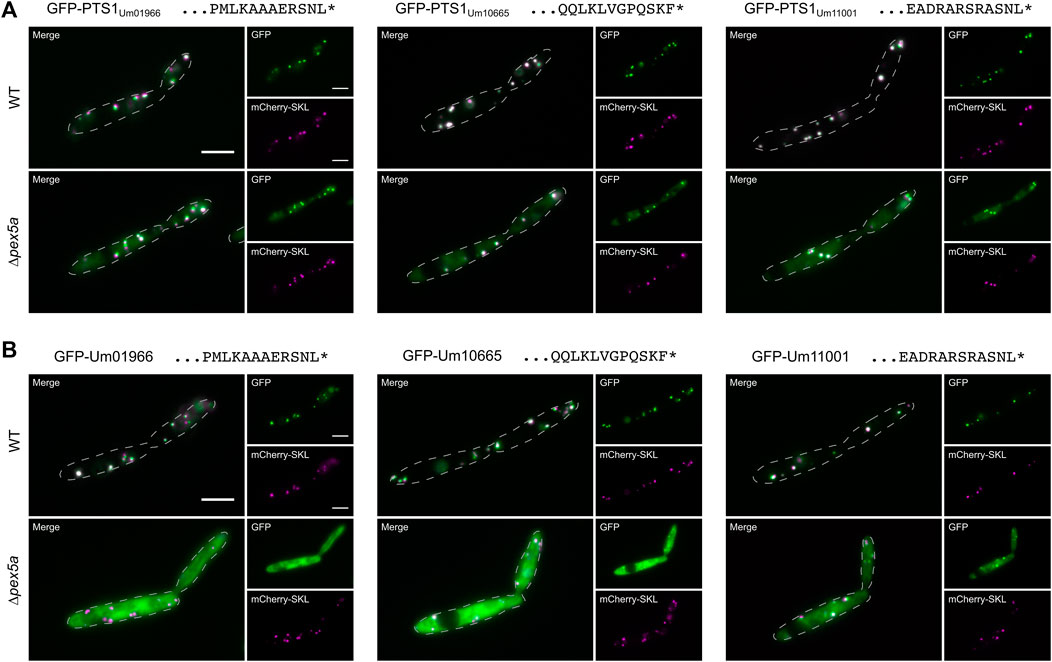
FIGURE 2. Identification of three cargo proteins of Pex5a. Fluorescence microscopic images of indicated strains expressing the peroxisomal marker protein mCherry-SKL (magenta) together with GFP (green) fused to C-terminal dodecamers including the PTS1 motifs of the U. maydis proteins Um01966, Um10665 and Um11001 (A), with GFP-tagged full-length version of these proteins (B). Scale bars represent 5 µm. These experiments show that localization of Um01966, Um10665 and Um11001 to peroxisomes is affected upon deletion of pex5a.
Um01966 is a putative acyl-CoA oxidase with high similarity to yeast Pox1, Um10665 a putative acyl-CoA dehydrogenase and Um11001 a putative enoyl-CoA hydratase (Table 1; Camões et al., 2015). N-terminally GFP-tagged full-length versions of all three proteins co-localized with mCherry-SKL in the presence of Pex5a, but showed pronounced cytosolic localization in ∆pex5a cells (Figure 2B). Cytosolic accumulation of GFP-tagged full-length proteins was even enhanced compared to the C-terminal dodecamers fused to GFP. Both experiments show that the three identified proteins are cargo with a preference for Pex5a.
Combinatorial Interaction With the NTD and the TPR Domain of Pex5a Determines Import Specificity
To discriminate between the function of the C-terminal TPR domain and the NTD of Pex5a for peroxisomal import of Um01966, Um10665 and Um11001 we added canonical SKL containing motifs at the C-terminus of each. Targeting to peroxisomes in ∆pex5a cells was drastically improved for GFP-Um10665-SKL and GFP-Um11001-SKL. Um01966-SKL predominantly co-localized with mCherry-SKL positive foci in wild type cells but substantial cytosolic mistargeting was observed in a strain deleted for pex5a (Figure 3A). This suggests a critical function of Pex5a for import of the acyl-CoA oxidase Um01966, which cannot be entirely bypassed by addition of a C-terminal canonical targeting signal.
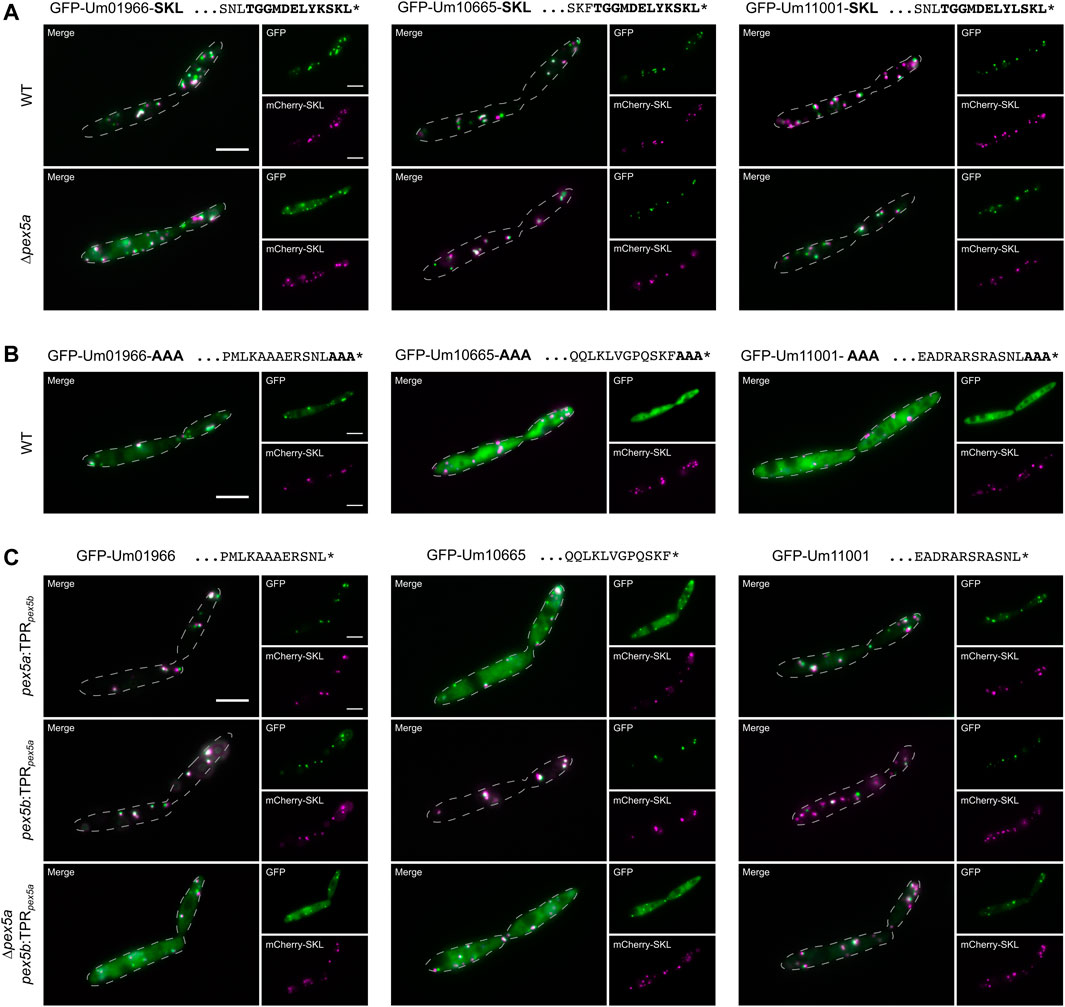
FIGURE 3. The NTD of Pex5a acts as targeting factor for Um01966. Fluorescence microscopic images of indicated strains expressing the peroxisomal marker protein mCherry-SKL (magenta) together with GFP-tagged full-length proteins (green) with their usual PTS1 masked by SKL (A) or AAA (B) in ∆pex5a and ∆pex5b cells. (C) Representative images of GFP-tagged full-length proteins expressed in mutants containing chimeric receptor proteins. These data reveal that both the NTD and the CTD contribute to cargo import.
Upon masking the PTS1 by C-terminal addition of three alanine residues (AAA), GFP-Um10665-AAA and GFP-Um11001-AAA remained largely cytosolic, while GFP-Um01966-AAA partially co-localized with mCherry-SKL (Figure 3B). A GFP-tagged and AAA-masked C-terminal dodecamer of Um01966 remained cytosolic revealing an additional targeting signal within the full-length protein (Supplementary Figure S5). Microscopic analysis of strains expressing chimeric variants of Pex5a and Pex5b demonstrated that the Pex5a TPRs are not required for efficient targeting of GFP-Um01966 to peroxisomes (Figure 3C). However, in the absence of the NTD of Pex5a (∆pex5a pex5b:TPRpex5a) residual peroxisomal targeting of GFP-Um01966 was observed (Figure 3C), while GFP-Um01966 localized almost entirely in the cytosol upon depletion of pex5a (Figure 2B). This is probably due to the presence of the Pex5a TPRs, which might partially compensate for the absence of the Pex5a NTD in ∆pex5a pex5b:TPRpex5a cells (Figure 3C). These results are in accordance with our initial observation that the isolated PTS1 containing sequence requires Pex5a for efficient import (Figure 2A). Targeting of GFP-Um10665 and to a minor extent GFP-Um11001 was reduced in the absence of each part of Pex5a (Figure 3C). Together, these experiments suggest that it is the combination of affinities to the NTD and the CTD of Pex5a, which facilitates recognition and import of Pex5a specific cargo. The impact of each of the two domains can be different depending on individual substrates.
PTS1 Motifs With Higher Affinity to the TPR Domains of Pex5a
To test the binding affinities of TPR domains of Pex5a and Pex5b (Figure 2), we set up a yeast two-hybrid experiment (Chien et al., 1991). We attached the TPR domains of either protein to the Gal4-DNA-binding domain (BD), and GFP with C-terminal dodecamers of different candidate proteins to the Gal4-activation domain (AD) (Figure 4A). As a control we used a C-terminal dodecamer, which efficiently mediates import of GFP into peroxisomes in the absence of Pex5a (Supplementary Figure S3; Um03158). Constructs were transformed in respective combinations into AH109∆pex5 (Stehlik et al., 2020). Interactions were monitored for three independent transformants per combination by growth assays on high stringency medium (Figure 4B). The assay revealed a stronger interaction of PTS1 motifs of Um01966, Um10665 and Um11001 with the TPR domain of Pex5a compared to the TPR domain of Pex5b. Among the tested candidates the PTS1 motif of Um03158 had the highest affinity to the TPRs of Pex5b and may thus be efficiently imported via both Pex5 proteins (Figure 4B). Interaction data from the two-hybrid experiment are in agreement with the data on import efficiency obtained by fluorescence microscopy (Figure 2). The weak interaction of the Um10665 PTS1 with the Pex5a TPRs may explain why efficient import of GFP-Um10665 is only observed when a native Pex5a protein containing the original NTD and CTD is expressed (Figure 3C). The strong interaction of the PTS1 of Um01966 with the Pex5a TPRs explains residual peroxisomal import of GFP-Um01966 in ∆pex5a pex5b:TPRpex5a cells (Figure 3C). Together, the results of the two-hybrid assay confirm that TPR domains of Pex5a and Pex5b show distinct preferences for variations of the C-terminal targeting signal and indicate that both cargo receptors have specific as well as shared substrates.
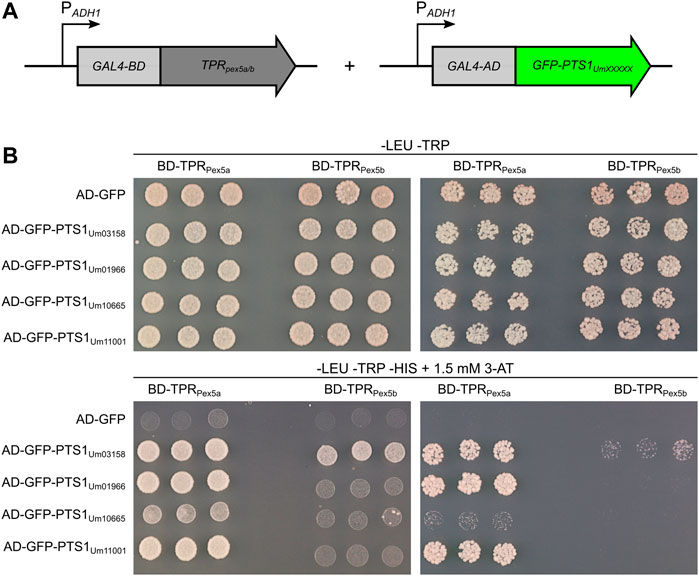
FIGURE 4. PTS1 motifs with higher affinity to Pex5a. (A) Schematic drawing of constructs used for the yeast two-hybrid assay. Constructs were expressed in a strain derived from AH109 deleted for PEX5 (Stehlik et al., 2020). TPR domains of either Pex5a or Pex5b were fused to the GAL4 DNA-binding domain (GAL4-BD), while C-terminal dodecamers of candidate proteins were fused to the GAL4 activation domain (GAL4-AD). (B) Fivefold and fiftyfold dilutions (OD600 = 0.2 and 0.02, respectively) of three independent transformants co-expressing indicated constructs were spotted on -LEU -TRP media and -LEU -TRP -HIS media and incubated for 3 days at 30°C. The selection medium (-LEU -TRP -HIS) contained 1.5 mM 3-Amino-1,2,4-triazole. This assay demonstrates that Um01966, Um10665 and Um11001 are substrates of the Pex5a TPRs and show only a very weak interaction with the Pex5b TPRs.
PTS1 Motifs With a Preference for Pex5b
Import of the putative Pox1 ortholog Um01966 shows similarity to Pox1 in S. cerevisiae since both can be imported via the NTD of a Pex5 protein (Klein et al., 2002). We wondered whether more similarities exist and tested import specificity of the glyoxylate cycle enzyme Mls1 – a Pex9 substrate in S. cerevisiae (Effelsberg et al., 2016; Yifrach et al., 2016) – in cells containing Pex5 derivatives with only one type of TPRs. To this end we fused the C-terminal dodecamers of the U. maydis malate synthase ortholog Mls1 (Um15004) to GFP. Indeed, we detected efficient peroxisomal import in the presence of the TPR domain of Pex5b but pronounced cytosolic localization when only the TPR domain of Pex5a was present (Figure 5A). mCherry-SKL predominantly localized in peroxisomes of both strains (Figure 5A). Mls1 from U. maydis contains the C-terminal tripeptide ARI. Remarkably, also a stop codon readthrough derived isoform of the glycolytic/gluconeogenetic enzyme triosephosphate isomerase (Tpi1; Um03299) harbors this C-terminal tripeptide (Freitag et al., 2012) and efficient import into peroxisomes also depends on the TPRs of Pex5b (Figure 5B). Thus, the highly similar PTS1 motifs of both enzymes are preferentially recognized by Pex5b.
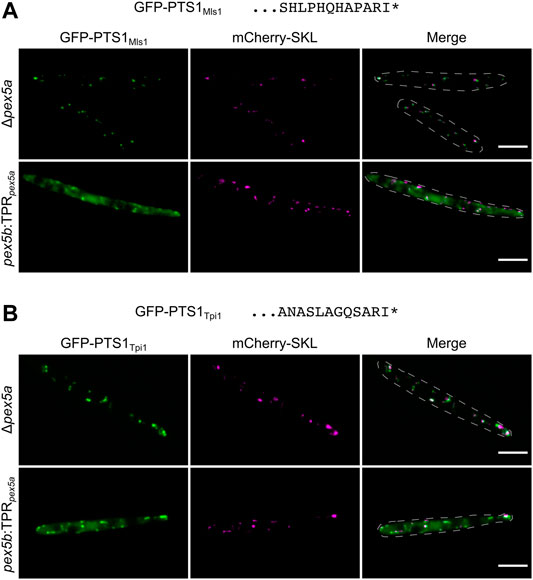
FIGURE 5. PTS1 motifs with a preference for Pex5b TPRs. Fluorescence microscopic images of indicated strains expressing the peroxisomal marker protein mCherry-SKL (magenta) together with GFP (green) fused to C-terminal dodecamers of Mls1 (malate synthase) (A) and Tpi1 (triosephosphate isomerase) (B). Both C-terminal dodecamers end on identical tripeptides and show efficient peroxisomal import when the TPR domains of Pex5b were present. Scale bars represent 5 µm.
Pex5b as Key Player for Matrix Protein Import in U. maydis
Of the cytosolic receptors, only Pex5b can mediate peroxisomal import as a single protein, while Pex5a requires the NTD of Pex5b (Figure 1). Pex5b might act as a co-receptor for Pex5a similar to Pex5-large for Pex7 in mammals or Pex18 and Pex21 for Pex7 in S. cerevisiae (Braverman et al., 1998; Otera et al., 1998; Purdue et al., 1998; Woodward and Bartel, 2005). Alternatively, Pex5b could be independently required for the activity of the peroxisomal import machinery.
In U. maydis PTS2-dependent transport may also involve Pex5b as a co-receptor, since the NTD of Pex5b contains a putative binding site for Pex7 (Figure 1; Kiel et al., 2006). In addition, the U. maydis genome lacks any ortholog of the yeast co-receptors Pex18 and Pex21 (Kämper et al., 2006). To test Pex5b for targeting of PTS2 proteins, we engineered a reporter protein by fusing an N-terminal fragment of the PTS2 protein Um01090 to GFP (PTS2-GFP; Figure 5A). The N-terminus of this predicted 3-keto-acyl-CoA thiolase related to yeast Pot1 contains a sequence matching the PTS2 consensus [R/K]-[L/V/I]-[X]5-[H/Q]-[L/A] (Lazarow, 2006; Camões et al., 2015; Kunze, 2020). We observed co-localization of PTS2-GFP with mCherry-SKL in peroxisomes and found that PTS2-GFP was retained in the cytosol upon deletion of the pex7 ortholog (um03596) (Figure 6A). To address whether Pex5b acts as co-receptor for Pex7, we expressed PTS2-GFP in pex5a and in pex5b deletion mutants. While peroxisomal localization was not affected in ∆pex5a cells, absence of pex5b completely abolished peroxisomal import of PTS2-GFP (Figure 6B). Reintroduction of the NTD of Pex5b into ∆pex5b mutants partially restored targeting of PTS2-GFP to peroxisomes suggesting that the NTD of Pex5b acts as co-receptor for Pex7 in U. maydis (Figure 6B).
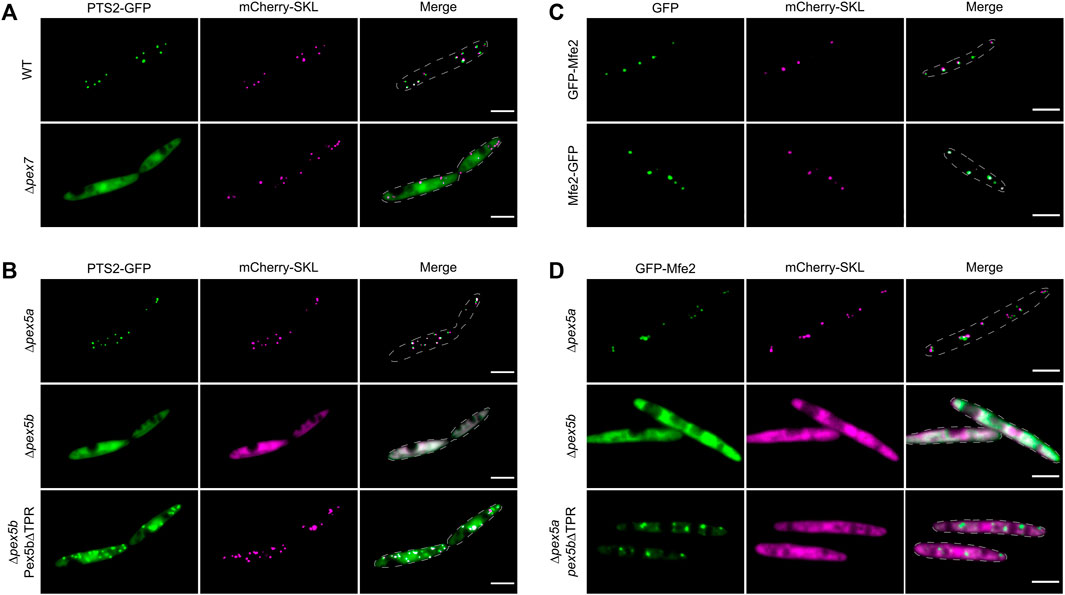
FIGURE 6. The NTD of Pex5b is a crucial factor for matrix protein import in U. maydis. Fluorescence microscopic images of indicated strains expressing mCherry-SKL (magenta) together with PTS2-GFP (green) (A,B) or with GFP-tagged Mfe2 (green) (C,D). The data show that the NTD of Pex5b is involved in peroxisomal targeting of PTS2 proteins and more unusual cargo such as the multifunctional enzymes (Mfe2). Scale bars represent 5 µm.
Pex5b-Dependent Import of a Matrix Protein Without a Canonical PTS
Finally, we focused on peroxisomal matrix proteins without a canonical peroxisomal targeting signal. Several proteins have been described that neither contain a PTS1 nor a PTS2 but, nevertheless, localize inside of peroxisomes (van der Klei and Veenhuis, 2006). In U. maydis, the multifunctional enzyme involved in peroxisomal fatty acid oxidation (Mfe2; Um10038) was characterized earlier (Klose and Kronstad, 2006). Although we could not detect any PTS motif in Mfe2, both N-terminally and C-terminally tagged GFP fusion proteins co-localized with mCherry-SKL suggesting that Mfe2 is imported into peroxisomes independently of recognition by Pex7 or the Pex5 TPRs (Figure 6C). We probed the mode of transport of Mfe2 by localization studies in a set of U. maydis mutants and found that peroxisomal targeting of Mfe2 requires Pex5b. The NTD of Pex5b was sufficient for partial peroxisomal localization of GFP-Mfe2 but import of Mfe2 was more efficient when the full-length protein was expressed (Figures 6C,D). This shows that the NTD of Pex5b also can act as receptor for peroxisomal matrix proteins in U. maydis.
Discussion
Our work uncovered a network of import modules for peroxisomal matrix proteins in U. maydis (Figure 7). We have identified five modules: Pex5b can transport substrates destined for the peroxisomal matrix either via its NTD (as coreceptor for Pex7 bound to PTS2-GFP, and Mfe2) or via its TPR domain (direct recognition of PTS1-containing proteins). The TPR domains of Pex5a and Pex5b bind to PTS1 motifs with overlapping but not identical specificity (Figures 2–5). The NTD of Pex5a also contributes to targeting but does not facilitate peroxisomal import in the absence of Pex5b (Figures 1–3, 7). Although the NTD of Pex5a is shorter in comparison to Pex5b, it contains the evolutionary conserved di-aromatic motifs for interaction with Pex14 (Figure 1). It is currently unclear why Pex5a alone is not sufficient to mediate cargo import. Recently, a role of Pex5 for insertion of membrane proteins was observed in S. cerevisiae (Martenson et al., 2020). A similar function of the Pex5b NTD may indirectly contribute to the critical importance of Pex5b for matrix protein import in U. maydis e.g., by targeting membrane proteins of the importomer.
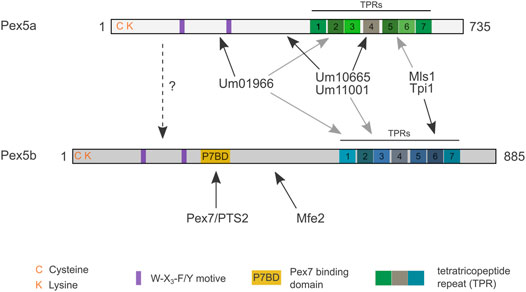
FIGURE 7. Model highlighting preferential cargo of the different domains of Pex5a and Pex5b. Cargo proteins of different domains are shown. Black arrows point to preferred binding domains. Gray arrows point to secondary binding sites. Please note that the cargo affinities of the TPRs are overlapping, while PTS2 import via Pex7 seems to be a specific function of Pex5b. For Mls1 and Tpi1 only specificity of the C-terminal dodecamer was determined. If an interaction between Pex5a and Pex5b can occur during import (dashed arrow) or if other functions of Pex5b determine its critical role for Pex5a-dependent import remains to be established.
Our genetic analyses demonstrate that two paralogs of Pex5 are necessary for optimal growth on oleic acid medium. Interestingly, key enzymes of the β-oxidation pathway seem to be preferentially targeted to peroxisomes via different factors. Pex5a is responsible for targeting of the acyl-CoA oxidase Um01966 related to S. cerevisiae Pox1, the multifunctional enzyme Mfe2 is imported into peroxisomes in the absence of Pex5a while the thiolase Um01090 depends on Pex7 for peroxisomal import (Figures 2, 6). Three different import routes may ensure the correct stoichiometry of enzymes inside of each peroxisome in particular when peroxisomes proliferate. Of interest, the S. cerevisiae ortholog Pox1 can bind to the NTD of Pex5 to target peroxisomes (Skoneczny and Lazarow, 1998; Klein et al., 2002). Malate synthase belongs to the cargo recognized by the Pex5 paralog Pex9 in S. cerevisae (Effelsberg et al., 2016; Yifrach et al., 2016; Yifrach et al., 2022). In U. maydis Mls1 is a preferred substrate of Pex5b. Thus, an evolutionary conservation of targeting mechanisms for particular peroxisomal proteins may exist indicating biological relevance of specific routes for specific enzymes.
In addition, we observed a growth defect on glucose medium for several of the pex5 mutants. A similar phenotype was already detected for U. maydis ∆pex3 and ∆pex6 cells as well as in other fungi (Idnurm et al., 2007; Freitag et al., 2012; Camões et al., 2015). Previously, we identified a metabolic network of carbohydrate metabolizing enzymes that are dually targeted to peroxisomes and the cytosol in a number of fungi (Freitag et al., 2012; Stiebler et al., 2014; Freitag et al., 2018; Kremp et al., 2020). A function of peroxisomes in regulating gluconeogenesis was recently described for S. cerevisiae and a number of novel, often dually localized peroxisomal proteins was discovered (Yifrach et al., 2021). Many metabolic and regulatory functions of peroxisomes still await elucidation and may contribute to reduced fitness observed for peroxisome-deficient mutants in glucose-containing medium. It is yet obscure, why growth of a mutant expressing only the NTD of Pex5b was more affected than any of the other strains (Figure 1). A possible explanation might be that import of only a subset of proteins e.g. Mfe2 or Pot1 is more detrimental for cells than retention of all peroxisomal matrix proteins in the cytosol.
Several peroxisomal proteins without a canonical PTS such as Mfe2 are known to bind to the NTD of Pex5 in S. cerevisiae (Skoneczny and Lazarow, 1998; Klein et al., 2002; Rymer et al., 2018; Kempiński et al., 2020). Aox1, Cta1, Fox2, Pox1 and Pox4 from different yeast species (Small et al., 1988; Kragler et al., 1993; Skoneczny and Lazarow, 1998; Gunkel et al., 2004; Rymer et al., 2018) resemble Um01966 and can be imported into peroxisomes if the PTS1 motifs are removed pointing to additional targeting signals. More such proteins likely exist, but two targeting signals may appear redundant and are therefore hard to detect. Specificity for a Pex5 protein seems to result from the combination of affinities towards the NTD and the CTD – for different cargo interaction with one or the other domain is more relevant or may even be sufficient (Figures 2–6). A very recent study focusing on TPR domains of the paralogs Pex5 and Pex9 from S. cerevisiae revealed that substrates can be discriminated by amino acids in vicinity of the C-terminal tripeptide (Yifrach et al., 2022). If this also applies to U. maydis or if other features of the motif determine specificity is an exciting question for future research. The C-terminal tripeptide could be important as well, as two of the three identified Pex5a substrates end on SNL, while two identified cargoes of Pex5b contain the tripeptide ARI at the C-terminus (Figures 2, 5).
Pex7-mediated import in U. maydis differs from several yeast species since specific co- receptors for PTS2 import are not present (Purdue et al., 1998; Schäfer et al., 2004; Kiel et al., 2006). We could show that in U. maydis PTS2 import depends on the NTD of Pex5b and this pathway shows more similarity to plants and mammals (Braverman et al., 1998; Otera et al., 1998; Woodward and Bartel, 2005; Kunze et al., 2015).
Allosteric interactions upon cargo binding are important for turning Pex5 and Pex7 into import-competent receptors attaching to the docking complex followed by translocation and cargo release (Kunze et al., 2015; Bürgi et al., 2021). The Pex5 CTD inhibits docking of the NTD in the absence of cargo; the NTD can translocate into the peroxisomal membrane when the CTD is deleted (Klein et al., 2002; Gouveia et al., 2003; Gunkel et al., 2004; Dias et al., 2017). In agreement with these data we found that overexpression of Pex5a lacking the TPRs can rescue the growth defect of ∆pex5a cells (Figure 1). Furthermore, we detected targeting of GFP-Mfe2 and PTS2-GFP to peroxisomes upon expression of the Pex5b NTD in the absence of the full-length protein (Figure 5). The capability of the peroxisomal import machinery to translocate large oligomeric cargo has been described (Walton et al., 1995; Yang et al., 2018). It will be interesting to establish how interactions at different sites of Pex5 proteins influence import of bigger complexes and import kinetics. In addition, the exact mechanistic function of both Pex5 proteins might be worth to evaluate.
Taken together, our study reveals the impact of different domains of Pex5 paralogs on cargo recognition and on peroxisome function in different growth conditions and contributes to a better understanding of peroxisomal protein import. Versatile import routes for matrix proteins seem to be widespread and may be critical for the formation of functional peroxisomes in many species.
Methods
Generation of Strains and Nucleic Acid Procedures
Constructs were generated using standard cloning procedures (Sambrook et al., 1989) or Gibson assembly (Gibson et al., 2009). All plasmids were verified by sequencing. Escherichia coli strain Top Ten (Invitrogen) was used for transformation according to a standard protocol (Hanahan et al., 1991) and propagation of plasmid DNA. Transformation of U. maydis cells was achieved as described previously (Schulz et al., 1990). Deletion strains and chimeric variants were created using an Sfi1 based cloning system (Brachmann et al., 2004; Kämper, 2004; Supplementary Figure S3). Genomic DNA was extracted according to an established protocol (Hoffman and Winston, 1987). Mutant strains were verified by Southern blot analysis (Sambrook et al., 1989). Proteins were expressed under control of the constitutive otef-promoter either integrated into the cbx-locus (Broomfield and Hargreaves, 1992; Spellig et al., 1996) or randomly integrated into the genome of U. maydis (mCherry-SKL). Similar expression levels were confirmed by measuring fluorescence using Synergy Mx multimode microplate reader (BioTek). All plasmids, strains and oligonucleotides used or generated during this study are listed in Supplementary Table S1. Progenitor plasmids used for this study were described (Spellig et al., 1996; Brachmann et al., 2004; Sandrock et al., 2006; Freitag et al., 2012; Stehlik et al., 2020). Genes can be accessed on NCBI.
Growth Conditions
U. maydis strains were grown at 28°C in liquid YEPSL (1% yeast extract, 0.4% peptone, 0.4% sucrose) or on solid potato dextrose broth containing 1.5% Bacto agar at 28°C. For selection solid media were supplemented with antibiotics (Brachmann et al., 2004). For growth assays 4 µl of serial tenfold dilutions of logarithmically growing cells starting with an OD600 of 1 (Figure 1B and Supplementary Figure S2) or 0.1 (Figure 1D) were spotted on solid minimal yeast nitrogen based media (Difco) with a pH of 5.7 supplemented with 0.5% ammonium sulfate. The plates contained 1.5% Bacto agar and either 2% glucose or a mixture of 0.2% oleic acid and 0.05% Tween-40. Plates were incubated for two (Figure 1B and Supplementary Figure S2) to 3 days (Figure 1D) at 23°C. All assays were at least repeated three times with similar results.
Growth Assays in Liquid Media
Cells of an OD600 of 1 were diluted to a starting OD600 of 0.1 and inoculated into fresh yeast nitrogen based media (Difco) with a pH of 5.7 supplemented with 0.5% ammonium sulfate and 2% glucose in a volume of 100 µl in flat bottom 96 well plates. Growth assays were performed in a Synergy Mx multimode microplate reader (BioTek) at 23°C with high shaking setting. OD600 was determined in 30 min intervals. Each strain was measured in five technical replicates and in three independent experiments. Mean values were plotted and original data and standard deviations are accessible in Supplementary Table S2.
Microscopy
A total of 200 µl of hot 1.5% agarose melted in water was used to create a thin agarose cushion on a 76 × 26 mm microscope slide (Roth). Cells from logarithmic growth phase incubated in yeast nitrogen based media (Difco) with a pH of 5.7 supplemented with 0.5% ammonium sulfate and 2% glucose were washed with water, concentrated fivefold, and 3 µl were spotted onto the middle of the agarose pad and covered with an 18 × 18 mm coverslip (Roth). Microscopy was performed on an Axiovert 200 M inverse microscope (Zeiss) equipped with a 1394 ORCA-ERA-CCD camera (Hamamatsu Photonics), filter sets for enhanced GFP (EGFP) and rhodamine (Chroma Technology), and a Zeiss 63×Plan Apochromat oil lens (NA 1.4). Single-plane bright field or phase contrast images and z-stacks of the cells (0.5 µm z-spacing) in the appropriate fluorescence channels were recorded, using the image acquisition software Volocity 5.3 (Perkin-Elmer). Images were processed and evaluated in ImageJ (Schneider et al., 2012). For protein localization analysis, single plain images or z-projections of deconvolved image stacks were used. Deconvolution was performed on the z-stacks by the ImageJ plugin DeconvolutionLab with 25 iterations of the Richardson – Lucy algorithm (Sage et al., 2017).
Yeast Two-Hybrid Assay
Sequences encoding the TPR domains of Pex5a and Pex5b were inserted into pGBKT7 (Matchmaker GAL4 Two-Hybrid System 3; Clontech) between the EcoRI and BamHI restriction sites via Gibson assembly. The ORFs for GFP or GFP modified with C-terminal dodecamers of Um01966, Um10665, Um11001, and Um03158 including PTS1 motifs were cloned into pGADT7 (Matchmaker GAL4 Two-Hybrid System 3; Clontech) between the EcoRI and BamHI restriction sites. Either pGBKT7-Pex5aTPR or pGBKT7-Pex5bTPR were co-transformed with one of the pGADT7 plasmids into YTS398, a derivative AH109 of deleted for pex5 (Stehlik et al., 2020). Three independent transformants of each of the 10 combinations were grown in liquid synthetic defined (SD) medium lacking leucine and tryptophan to an OD600 of approx. 1. Cells were washed once with sterile water and 4 µl of fivefold or fiftyfold dilutions (OD600 = 0.2 or 0.02) were spotted on solid SD medium lacking leucine and tryptophan as growth control, and on SD medium lacking leucine, tryptophan and histidine, and containing 1.5 mM 3-amino-1,2,4-triazole to test for protein – protein interaction. Plates were incubated for 3 days at 30°C.
Data Availability Statement
The original contributions presented in the study are included in the article/Supplementary Material, further inquiries can be directed to the corresponding author.
Author Contributions
JA, MB, and JF designed the study. JA, NB, EB, DM, and HA performed the experiments. All authors contributed to data analysis. JA, NB, and EB created the figures. JF wrote the manuscript with input from all authors.
Conflict of Interest
The authors declare that the research was conducted in the absence of any commercial or financial relationships that could be construed as a potential conflict of interest.
Publisher’s Note
All claims expressed in this article are solely those of the authors and do not necessarily represent those of their affiliated organizations, or those of the publisher, the editors and the reviewers. Any product that may be evaluated in this article, or claim that may be made by its manufacturer, is not guaranteed or endorsed by the publisher.
Acknowledgments
We acknowledge Marisa Piscator for excellent technical assistance and Regine Kahmann from the MPI for Terrestrial Microbiology for sharing facilities. JA was supported by a fellowship from the Marburg Research Academy. MB acknowledges funding from DFG (BO2094-5). JF was supported by the DFG (grant ID FR-3586/2-1). We thank Thorsten Stehlik for many discussions and Björn Sandrock for critical reading of the manuscript. We are grateful to Michael Feldbrügge and Jörg Kämper for sharing plasmids. Open Access funding was provided by the Open Acess Publication Fund of Philipps-Universität Marburg with support of the DFG.
Supplementary Material
The Supplementary Material for this article can be found online at: https://www.frontiersin.org/articles/10.3389/fcell.2022.858084/full#supplementary-material
References
Amery, L., Sano, H., Mannaerts, G. P., Snider, J., van Looy, J., Fransen, M., et al. (2001). Identification of PEX5p-Related Novel Peroxisome-Targeting Signal 1 (PTS1)-Binding Proteins in Mammals. Biochem. J. 357, 635–646. doi:10.1042/bj3570635
Brachmann, A., König, J., Julius, C., and Feldbrügge, M. (2004). A Reverse Genetic Approach for Generating Gene Replacement Mutants in Ustilago maydis. Mol. Genet. Genomics 272, 216–226. doi:10.1007/s00438-004-1047-z
Braverman, N., Dodt, G., Gould, S. J., and Valle, D. (1998). An Isoform of Pex5p, the Human PTS1 Receptor, Is Required for the Import of PTS2 Proteins into Peroxisomes. Hum. Mol. Genet. 7, 1195–1205. doi:10.1093/hmg/7.8.1195
Braverman, N., Steel, G., Obie, C., Moser, A., Moser, H., Gould, S. J., et al. (1997). Human PEX7 Encodes the Peroxisomal PTS2 Receptor and Is Responsible for Rhizomelic Chondrodysplasia Punctata. Nat. Genet. 15, 369–376. doi:10.1038/ng0497-369
Brocard, C., and Hartig, A. (2006). Peroxisome Targeting Signal 1: Is it Really a Simple Tripeptide? Biochim. Biophys. Acta (Bba) - Mol. Cel. Res. 1763, 1565–1573. doi:10.1016/j.bbamcr.2006.08.022
Brocard, C., Kragler, F., Simon, M. M., Schuster, T., and Hartig, A. (1994). The Tetratricopeptide Repeat Domain of the PAS10 Protein of Saccharomyces Cerevisiae Is Essential for Binding the Peroxisomal Targeting Signal-SKL. Biochem. Biophysical Res. Commun. 204, 1016–1022. doi:10.1006/bbrc.1994.2564
Broomfield, P. L. E., and Hargreaves, J. A. (1992). A Single Amino-Acid Change in the Iron-Sulphur Protein Subunit of Succinate Dehydrogenase Confers Resistance to Carboxin in Ustilago maydis. Curr. Genet. 22, 117–121. doi:10.1007/bf00351470
Bürgi, J., Ekal, L., and Wilmanns, M. (2021). Versatile Allosteric Properties in Pex5-Like Tetratricopeptide Repeat Proteins to Induce Diverse Downstream Function. Traffic 22, 140–152. doi:10.1111/tra.12785
Camões, F., Islinger, M., Guimarães, S. C., Kilaru, S., Schuster, M., Godinho, L. F., et al. (2015). New Insights into the Peroxisomal Protein Inventory: Acyl-CoA Oxidases And-Dehydrogenases Are an Ancient Feature of Peroxisomes. Biochim. Biophys. Acta (Bba)-Molecular Cel. Res. 1853, 111–125. doi:10.1016/j.bbamcr.2014.10.005
Carvalho, A. F., Costa-Rodrigues, J., Correia, I., Costa Pessoa, J., Faria, T. Q., Martins, C. L., et al. (2006). The N-Terminal Half of the Peroxisomal Cycling Receptor Pex5p Is a Natively Unfolded Domain. J. Mol. Biol. 356, 864–875. doi:10.1016/j.jmb.2005.12.002
Chien, C. T., Bartel, P. L., Sternglanz, R., and Fields, S. (1991). The Two-Hybrid System: a Method to Identify and Clone Genes for Proteins that Interact with a Protein of Interest. Proc. Natl. Acad. Sci. U.S.A. 88, 9578–9582. doi:10.1073/pnas.88.21.9578
Dias, A. F., Rodrigues, T. A., Pedrosa, A. G., Barros-Barbosa, A., Francisco, T., and Azevedo, J. E. (2017). The Peroxisomal Matrix Protein Translocon Is a Large Cavity-Forming Protein Assembly into Which PEX5 Protein Enters to Release its Cargo. J. Biol. Chem. 292, 15287–15300. doi:10.1074/jbc.m117.805044
Edward Purdue, P., Yang, X., and Lazarow, P. B. (1998). Pex18p and Pex21p, a Novel Pair of Related Peroxins Essential for Peroxisomal Targeting by the PTS2 Pathway. J. Cel. Biol. 143, 1859–1869. doi:10.1083/jcb.143.7.1859
Effelsberg, D., Cruz-Zaragoza, L. D., Schliebs, W., and Erdmann, R. (2016). Pex9p Is a New Yeast Peroxisomal Import Receptor for PTS1-Containing Proteins. J. Cel. Sci. 129, 4057–4066. doi:10.1242/jcs.195271
Effelsberg, D., Cruz-Zaragoza, L. D., Tonillo, J., Schliebs, W., and Erdmann, R. (2015). Role of Pex21p for Piggyback Import of Gpd1p and Pnc1p into Peroxisomes of Saccharomyces cerevisiae. J. Biol. Chem. 290, 25333–25342. doi:10.1074/jbc.m115.653451
El Magraoui, F., Brinkmeier, R., Mastalski, T., Hupperich, A., Strehl, C., Schwerter, D., et al. (2019). The Deubiquitination of the PTS1-Import Receptor Pex5p Is Required for Peroxisomal Matrix Protein Import. Biochim. Biophys. Acta (Bba) - Mol. Cel. Res. 1866, 199–213. doi:10.1016/j.bbamcr.2018.11.002
Francisco, T., Rodrigues, T. A., Dias, A. F., Barros-Barbosa, A., Bicho, D., and Azevedo, J. E. (2017). Protein Transport into Peroxisomes: Knowns and Unknowns. BioEssays 39, 1700047. doi:10.1002/bies.201700047
Freitag, J., Ast, J., and Bölker, M. (2012). Cryptic Peroxisomal Targeting via Alternative Splicing and Stop Codon Read-Through in Fungi. Nature 485, 522–525. doi:10.1038/nature11051
Freitag, J., Stehlik, T., Stiebler, A. C., and Bölker, M. (2018). “The Obvious and the Hidden: Prediction and Function of Fungal Peroxisomal Matrix Proteins,” in Proteomics of Peroxisomes. Springer, 139–155.
Gabaldón, T. (2010). Peroxisome Diversity and Evolution. Philos. Trans. R. Soc. Lond. B. Biol. Sci. 365, 765–773. doi:10.1098/rstb.2009.0240
Gabay-Maskit, S., Cruz-Zaragoza, L. D., Shai, N., Eisenstein, M., Bibi, C., Cohen, N., et al. (2020). A Piggybacking Mechanism Enables Peroxisomal Localization of the Glyoxylate Cycle Enzyme Mdh2 in Yeast. J. Cell Sci. 133, jcs244376. doi:10.1242/jcs.244376
Gardner, B. M., Castanzo, D. T., Chowdhury, S., Stjepanovic, G., Stefely, M. S., Hurley, J. H., et al. (2018). The Peroxisomal AAA-ATPase Pex1/Pex6 Unfolds Substrates by Processive Threading. Nat. Commun. 9, 135. doi:10.1038/s41467-017-02474-4
Gatto, G. J., Geisbrecht, B. V., Gould, S. J., and Berg, J. M. (2000). Peroxisomal Targeting Signal-1 Recognition by the TPR Domains of Human PEX5. Nat. Struct. Biol. 7, 1091–1095. doi:10.1038/81930
Gibson, D. G., Young, L., Chuang, R.-Y., Venter, J. C., Hutchison, C. A., and Smith, H. O. (2009). Enzymatic Assembly of DNA Molecules up to Several Hundred Kilobases. Nat. Methods 6, 343–345. doi:10.1038/nmeth.1318
Glover, J. R., Andrews, D. W., and Rachubinski, R. A. (1994). Saccharomyces Cerevisiae Peroxisomal Thiolase is Imported as a Dimer. Proc. Natl. Acad. Sci. 91, 10541–10545.
Gould, S. J., Kalish, J. E., Morrell, J. C., Bjorkman, J., Urquhart, A. J., and Crane, D. I. (1996). Pex13p Is an SH3 Protein of the Peroxisome Membrane and a Docking Factor for the Predominantly Cytoplasmic PTs1 Receptor. J. Cel. Biol. 135, 85–95. doi:10.1083/jcb.135.1.85
Gould, S. J., Keller, G. A., Hosken, N., Wilkinson, J., and Subramani, S. (1989). A Conserved Tripeptide Sorts Proteins to Peroxisomes. J. Cel. Biol. 108, 1657–1664. doi:10.1083/jcb.108.5.1657
Gouveia, A. M., Guimarães, C. P., Oliveira, M. E., Sá-Miranda, C., and Azevedo, J. E. (2003). Insertion of Pex5p into the Peroxisomal Membrane Is Cargo Protein-Dependent. J. Biol. Chem. 278, 4389–4392. doi:10.1074/jbc.c200650200
Gunkel, K., van Dijk, R., Veenhuis, M., and van der Klei, I. J. (2004). Routing of Hansenula polymorpha Alcohol Oxidase: An Alternative Peroxisomal Protein-Sorting Machinery. MBoC 15, 1347–1355. doi:10.1091/mbc.e03-04-0258
Hanahan, D., Jessee, J., and Bloom, F. R. (1991). [4] Plasmid Transformation of Escherichia coli and Other Bacteria. Methods Enzymol. 204, 63–113. doi:10.1016/0076-6879(91)04006-a
Hoffman, C. S., and Winston, F. (1987). A Ten-Minute DNA Preparation from Yeast Efficiently Releases Autonomous Plasmids for Transformaion of Escherichia coli. Gene 57, 267–272. doi:10.1016/0378-1119(87)90131-4
Idnurm, A., Giles, S. S., Perfect, J. R., and Heitman, J. (2007). Peroxisome Function Regulates Growth on Glucose in the Basidiomycete Fungus Cryptococcus Neoformans. Eukaryot. Cel. 6, 60–72. doi:10.1128/ec.00214-06
Islinger, M., Li, K. W., Seitz, J., Völkl, A., and Lüers, G. H. (2009). Hitchhiking of Cu/Zn Superoxide Dismutase to Peroxisomes - Evidence for a Natural Piggyback Import Mechanism in Mammals. Traffic 10, 1711–1721. doi:10.1111/j.1600-0854.2009.00966.x
Kämper, J. (2004). A PCR-Based System for Highly Efficient Generation of Gene Replacement Mutants in Ustilago maydis. Mol. Genet. Genomics 271, 103–110. doi:10.1007/s00438-003-0962-8
Kämper, J., Kahmann, R., Bölker, M., Ma, L. J., Brefort, T., Saville, B. J., et al. (2006). Insights from the Genome of the Biotrophic Fungal Plant Pathogen Ustilago maydis. Nature 444, 97–101. doi:10.1038/nature05248
Kempiński, B., Chełstowska, A., Poznański, J., Król, K., Rymer, Ł., Frydzińska, Z., et al. (2020). The Peroxisomal Targeting Signal 3 (PTS3) of the Budding Yeast Acyl-CoA Oxidase Is a Signal Patch. Front. Cel. Dev. Biol. 8, 198. doi:10.3389/fcell.2020.00198
Kiel, J. A. K. W., Veenhuis, M., and Van Der Klei, I. J. (2006). PEX Genes in Fungal Genomes: Common, Rare or Redundant. Traffic 7, 1291–1303. doi:10.1111/j.1600-0854.2006.00479.x
Kim, P. K., and Hettema, E. H. (2015). Multiple Pathways for Protein Transport to Peroxisomes. J. Mol. Biol. 427, 1176–1190. doi:10.1016/j.jmb.2015.02.005
Klein, A. T. J., van den Berg, M., Bottger, G., Tabak, H. F., and Distel, B. (2002). Saccharomyces cerevisiae Acyl-CoA Oxidase Follows a Novel, Non-PTS1, Import Pathway into Peroxisomes that Is Dependent on Pex5p. J. Biol. Chem. 277, 25011–25019. doi:10.1074/jbc.m203254200
Klose, J., and Kronstad, J. W. (2006). The Multifunctional β-Oxidation Enzyme Is Required for Full Symptom Development by the Biotrophic Maize Pathogen Ustilago maydis. Eukaryot. Cel. 5, 2047–2061. doi:10.1128/ec.00231-06
Kragler, F., Langeder, A., Raupachova, J., Binder, M., and Hartig, A. (1993). Two Independent Peroxisomal Targeting Signals in Catalase A of Saccharomyces cerevisiae. J. Cel. Biol. 120, 665–673. doi:10.1083/jcb.120.3.665
Kremp, M., Bittner, E., Martorana, D., Klingenberger, A., Stehlik, T., Bölker, M., et al. (2020). Non-AUG Translation Initiation Generates Peroxisomal Isoforms of 6-Phosphogluconate Dehydrogenase in Fungi. Front. Cel. Dev. Biol. 8, 251. doi:10.3389/fcell.2020.00251
Kunze, M., Malkani, N., Maurer-Stroh, S., Wiesinger, C., Schmid, J. A., and Berger, J. (2015). Mechanistic Insights into PTS2-Mediated Peroxisomal Protein Import. J. Biol. Chem. 290, 4928–4940. doi:10.1074/jbc.m114.601575
Kunze, M., Neuberger, G., Maurer-Stroh, S., Ma, J., Eck, T., Braverman, N., et al. (2011). Structural Requirements for Interaction of Peroxisomal Targeting Signal 2 and its Receptor PEX7. J. Biol. Chem. 286, 45048–45062. doi:10.1074/jbc.m111.301853
Kunze, M. (2020). The Type-2 Peroxisomal Targeting Signal. Biochim. Biophys. Acta (Bba) - Mol. Cel. Res. 1867, 118609. doi:10.1016/j.bbamcr.2019.118609
Lanver, D., Tollot, M., Schweizer, G., Lo, P. L., Reissmann, S., Ma, L.-S., et al. (2017). Ustilago Maydis Effectors and Their Impact on Virulence. Nat. Rev. Microbiol. 15, 409.
Lazarow, P. B. (2006). Chapter 3.1.7. The Import Receptor Pex7p and the PTS2 Targeting Sequence. Biochim. Biophys. Acta (Bba) - Mol. Cel. Res. 1763, 1599–1604. doi:10.1016/j.bbamcr.2006.08.011
Lill, P., Hansen, T., Wendscheck, D., Klink, B. U., Jeziorek, T., Vismpas, D., et al. (2020). Towards the Molecular Architecture of the Peroxisomal Receptor Docking Complex. Proc. Natl. Acad. Sci. U.S.A. 117, 33216–33224. doi:10.1073/pnas.2009502117
Lingner, T., Kataya, A. R., Antonicelli, G. E., Benichou, A., Nilssen, K., Chen, X.-Y., et al. (2011). Identification of Novel Plant Peroxisomal Targeting Signals by a Combination of Machine Learning Methods and In Vivo Subcellular Targeting Analyses. Plant Cell 23, 1556–1572. doi:10.1105/tpc.111.084095
Lodish, H., Berk, A., Zipursky, S. L., Matsudaira, P., Baltimore, D., and Darnell, J. (2000). “Organelles of the Eukaryotic Cell,” in Molecular Cell Biology. 4th edition. New York: W.H. Freeman.
Martenson, J. S., Tam, H., McQuown, A. J., Reif, D., Zhou, J., and Denic, V. (2020). The Importomer Is a Peroxisomal Membrane Protein Translocase. bioRxiv.
McNew, J. A., and Goodman, J. M. (1994). An Oligomeric Protein is Imported into Peroxisomes in vivo. J. Cell Biol. 127, 1245–1257.
Meinecke, M., Cizmowski, C., Schliebs, W., Krüger, V., Beck, S., Wagner, R., et al. (2010). The Peroxisomal Importomer Constitutes a Large and Highly Dynamic Pore. Nat. Cel. Biol. 12, 273–277. doi:10.1038/ncb2027
Miyata, N., and Fujiki, Y. (2005). Shuttling Mechanism of Peroxisome Targeting Signal Type 1 Receptor Pex5: ATP-Independent Import and ATP-Dependent Export. Mol. Cel. Biol. 25, 10822–10832. doi:10.1128/mcb.25.24.10822-10832.2005
Nötzel, C., Lingner, T., Klingenberg, H., and Thoms, S. (2016). Identification of New Fungal Peroxisomal Matrix Proteins and Revision of the PTS1 Consensus. Traffic 17, 1110–1124. doi:10.1111/tra.12426
Otera, H., Okumoto, K., Tateishi, K., Ikoma, Y., Matsuda, E., Nishimura, M., et al. (1998). Peroxisome Targeting Signal Type 1 (PTS1) Receptor Is Involved in Import of Both PTS1 and PTS2: Studies with PEX5-Defective CHO Cell Mutants. Mol. Cel. Biol. 18, 388–399. doi:10.1128/mcb.18.1.388
Otera, H., Setoguchi, K., Hamasaki, M., Kumashiro, T., Shimizu, N., and Fujiki, Y. (2002). Peroxisomal Targeting Signal Receptor Pex5p Interacts with Cargoes and Import Machinery Components in a Spatiotemporally Differentiated Manner: Conserved Pex5p WXXXF/Y Motifs Are Critical for Matrix Protein Import. Mol. Cel. Biol. 22, 1639–1655. doi:10.1128/mcb.22.6.1639-1655.2002
Pedrosa, A. G., Francisco, T., Bicho, D., Dias, A. F., Barros-Barbosa, A., Hagmann, V., et al. (2018). Peroxisomal Monoubiquitinated PEX5 Interacts with the AAA ATPases PEX1 and PEX6 and Is Unfolded During its Dislocation into the Cytosol. J. Biol. Chem. 293, 11553–11563. doi:10.1074/jbc.ra118.003669
Platta, H. W., Grunau, S., Rosenkranz, K., Girzalsky, W., and Erdmann, R. (2005). Functional Role of the AAA Peroxins in Dislocation of the Cycling PTS1 Receptor Back to the Cytosol. Nat. Cel. Biol. 7, 817–822. doi:10.1038/ncb1281
Platta, H. W., Magraoui, F. E., Schlee, D., Grunau, S., Girzalsky, W., and Erdmann, R. (2007). Ubiquitination of the Peroxisomal Import Receptor Pex5p Is Required for its Recycling. J. Cel. Biol. 177, 197–204. doi:10.1083/jcb.200611012
Rosenthal, M., Metzl-Raz, E., Bürgi, J., Yifrach, E., Drwesh, L., Fadel, A., et al. (2020). Uncovering Targeting Priority to Yeast Peroxisomes Using an In-Cell Competition Assay. Proc. Natl. Acad. Sci. U.S.A. 117, 21432–21440. doi:10.1073/pnas.1920078117
Rymer, Ł., Kempiński, B., Chełstowska, A., and Skoneczny, M. (2018). The Budding Yeast Pex5p Receptor Directs Fox2p and Cta1p into Peroxisomes via its N-Terminal Region Near the FxxxW Domain. J. Cel. Sci. 131, jcs216986. doi:10.1242/jcs.216986
Sage, D., Donati, L., Soulez, F., Fortun, D., Schmit, G., Seitz, A., et al. (2017). DeconvolutionLab2: An Open-Source Software for Deconvolution Microscopy. Methods 115, 28–41. doi:10.1016/j.ymeth.2016.12.015
Saidowsky, J., Dodt, G., Kirchberg, K., Wegner, A., Nastainczyk, W., Kunau, W.-H., et al. (2001). The Di-Aromatic Pentapeptide Repeats of the Human Peroxisome Import Receptor PEX5 Are Separate High Affinity Binding Sites for the Peroxisomal Membrane Protein PEX14. J. Biol. Chem. 276, 34524–34529. doi:10.1074/jbc.m104647200
Sambrook, J., Fritsch, E. F., and Maniatis, T. (1989). in Molecular Cloning: A Laboratory Manual. Editor J. Argentine. (Cold Spring Harbor, NY: Cold Spring Harbor Laboratory Press).
Sandrock, B., Böhmer, C., and Bölker, M. (2006). Dual Function of the Germinal Centre Kinase Don3 During Mitosis and Cytokinesis in Ustilago maydis. Mol. Microbiol. 62, 655–666. doi:10.1111/j.1365-2958.2006.05405.x
Santoro, B., Hu, L., Liu, H., Saponaro, A., Pian, P., Piskorowski, R. A., et al. (2011). TRIP8b Regulates HCN1 Channel Trafficking and Gating Through Two Distinct C-Terminal Interaction Sites. J. Neurosci. 31, 4074–4086. doi:10.1523/jneurosci.5707-10.2011
Santoro, B., Wainger, B. J., and Siegelbaum, S. A. (2004). Regulation of HCN Channel Surface Expression by a Novel C-Terminal Protein-Protein Interaction. J. Neurosci. 24, 10750–10762. doi:10.1523/jneurosci.3300-04.2004
Saryi, N. A. A., Hutchinson, J. D., Al-hejjaj, M. Y., Sedelnikova, S., Baker, P., and Hettema, E. H. (2017). Pnc1 Piggy-Back Import into Peroxisomes Relies on Gpd1 Homodimerisation. Sci. Rep. 7, 42579. doi:10.1038/srep42579
Schäfer, A., Kerssen, D., Veenhuis, M., Kunau, W. H., and Schliebs, W. (2004). Functional Similarity Between the Peroxisomal PTS2 Receptor Binding Protein Pex18p and the N-Terminal Half of the PTS1 Receptor Pex5p. Mol. Cel. Biol. 24, 8895–8906. doi:10.1128/MCB.24.20.8895-8906.2004
Schneider, C. A., Rasband, W. S., and Eliceiri, K. W. (2012). NIH Image to ImageJ: 25 Years of Image Analysis. Nat. Methods 9, 671–675. doi:10.1038/nmeth.2089
Schueren, F., Lingner, T., George, R., Hofhuis, J., Dickel, C., Gärtner, J., et al. (2014). Peroxisomal Lactate Dehydrogenase Is Generated by Translational Readthrough in Mammals. Elife 3, e03640. doi:10.7554/eLife.03640
Schulz, B., Banuett, F., Dahl, M., Schlesinger, R., Schäfer, W., Martin, T., et al. (1990). The B Alleles of U. Maydis, Whose Combinations Program Pathogenic Development, Code for Polypeptides Containing a Homeodomain-Related Motif. Cell 60, 295–306. doi:10.1016/0092-8674(90)90744-y
Skoneczny, M., and Lazarow, P. B. (1998). A Novel, Non-PTS1, Peroxisomal Import Route Dependent on the PTS1 Receptor Pex5p. Mol. Biol. Cel. 348A.
Small, G. M., Szabo, L. J., and Lazarow, P. B. (1988). Acyl-CoA Oxidase Contains Two Targeting Sequences Each of Which Can Mediate Protein Import into Peroxisomes. EMBO J. 7, 1167–1173. doi:10.1002/j.1460-2075.1988.tb02927.x
Smith, J. J., and Aitchison, J. D. (2013). Peroxisomes Take Shape. Nat. Rev. Mol. Cel. Biol. 14, 803–817. doi:10.1038/nrm3700
Spellig, T., Bottin, A., and Kahmann, R. (1996). Green Fluorescent Protein (GFP) as a New Vital Marker in the Phytopathogenic Fungus Ustilago maydis. Mol. Gen. Genet. 252, 503–509. doi:10.1007/s004380050257
Stiebler, A. C., Freitag, J., Schink, K. O., Tillmann, B. A. M., Ast, J., and Bölker, M. (2014). Ribosomal Readthrough at a Short UGA Stop Codon Context Triggers Dual Localization of Metabolic Enzymes in Fungi and Animals. PLOS Genet. 10, e1004685.
Stehlik, T., Kremp, M., Kahnt, J., Bölker, M., and Freitag, J. (2020). Peroxisomal Targeting of a Protein Phosphatase Type 2C via Mitochondrial Transit. Nat. Commun. 11, 2355. doi:10.1038/s41467-020-16146-3
Su, J.-R., Takeda, K., Tamura, S., Fujiki, Y., and Miki, K. (2009). Crystal Structure of the Conserved N-Terminal Domain of the Peroxisomal Matrix Protein Import Receptor, Pex14p. Proc. Natl. Acad. Sci. U.S.A. 106, 417–421. doi:10.1073/pnas.0808681106
Urquhart, A. J., Kennedy, D., Gould, S. J., and Crane, D. I. (2000). Interaction of Pex5p, the Type 1 Peroxisome Targeting Signal Receptor, with the Peroxisomal Membrane Proteins Pex14p and Pex13p. J. Biol. Chem. 275, 4127–4136. doi:10.1074/jbc.275.6.4127
van der Klei, I. J., and Veenhuis, M. (2006). PTS1-Independent Sorting of Peroxisomal Matrix Proteins by Pex5p. Biochim. Biophys. Acta (Bba) - Mol. Cel. Res. 1763, 1794–1800. doi:10.1016/j.bbamcr.2006.08.013
Walter, T., and Erdmann, R. (2019). Current Advances in Protein Import into Peroxisomes. Protein J. 38, 351–362. doi:10.1007/s10930-019-09835-6
Walton, P. A., Hill, P. E., and Subramani, S. (1995). Import of Stably Folded Proteins into Peroxisomes. Mol. Biol. Cel. 6, 675–683. doi:10.1091/mbc.6.6.675
Wanders, R. J. A. (2014). Metabolic Functions of Peroxisomes in Health and Disease. Biochimie 98, 36–44. doi:10.1016/j.biochi.2013.08.022
Woodward, A. W., and Bartel, B. (2005). The Arabidopsis Peroxisomal Targeting Signal Type 2 Receptor PEX7 Is Necessary for Peroxisome Function and Dependent on PEX5. MBoC 16, 573–583. doi:10.1091/mbc.e04-05-0422
Yang, J., Pieuchot, L., and Jedd, G. (2018). Artificial Import Substrates Reveal an Omnivorous Peroxisomal Importomer. Traffic 19, 786–797. doi:10.1111/tra.12607
Yifrach, E., Chuartzman, S. G., Dahan, N., Maskit, S., Zada, L., Weill, U., et al. (2016). Characterization of Proteome Dynamics During Growth in Oleate Reveals a New Peroxisome-Targeting Receptor. J. Cel. Sci. 129, 4067–4075. doi:10.1242/jcs.195255
Yifrach, E., Holbrook-Smith, D., Bürgi, J., Othman, A., Eisenstein, M., Van Roermund, C. W. T., et al. (2021). Systematic Multi-Level Analysis of an Organelle Proteome Reveals New Peroxisomal Functions. bioRxiv. doi:10.1101/2021.12.08.471723
Keywords: PEX5, PEX7, beta oxidation, peroxisome, targeting signal, Ustilago maydis, PTS1, PTS2
Citation: Ast J, Bäcker N, Bittner E, Martorana D, Ahmad H, Bölker M and Freitag J (2022) Two Pex5 Proteins With Different Cargo Specificity Are Critical for Peroxisome Function in Ustilago maydis. Front. Cell Dev. Biol. 10:858084. doi: 10.3389/fcell.2022.858084
Received: 19 January 2022; Accepted: 31 March 2022;
Published: 12 May 2022.
Edited by:
Marek Skoneczny, Institute of Biochemistry and Biophysics (PAN), PolandReviewed by:
Jorge E. Azevedo, Universidade do Porto, PortugalMarkus Kunze, Medical University of Vienna, Austria
Copyright © 2022 Ast, Bäcker, Bittner, Martorana, Ahmad, Bölker and Freitag. This is an open-access article distributed under the terms of the Creative Commons Attribution License (CC BY). The use, distribution or reproduction in other forums is permitted, provided the original author(s) and the copyright owner(s) are credited and that the original publication in this journal is cited, in accordance with accepted academic practice. No use, distribution or reproduction is permitted which does not comply with these terms.
*Correspondence: Johannes Freitag, johannes.freitag@biologie.uni-marburg.de