A bioflocculant from Corynebacterium glutamicum and its application in acid mine wastewater treatment
- 1Tianjin Key Laboratory of Industrial Microbiology, College of Biotechnology, Tianjin University of Science and Technology, Tianjin, China
- 2National Engineering Laboratory for Industrial Enzymes, Tianjin Institute of Industrial Biotechnology, Chinese Academy of Sciences, Tianjin, China
Although many microorganisms have been found to produce bioflocculants, and bioflocculants have been considered as attractive alternatives to chemical flocculants in wastewater treatment, there are few reports on bioflocculants from the safe strain C. glutamicum, and the application of bioflocculants in acid wastewater treatment is also rare attributed to the high content of metal ions and high acidity of the water. In this study, a novel bioflocculant produced by Corynebacterium glutamicum Cg1-P30 was investigated. An optimal production of this bioflocculant with a yield of 0.52 g/L was achieved by Box–Behnken design, using 12.20 g/L glucose, 4.00 g/L corn steep liquor and 3.60 g/L urea as carbon and nitrogen source. The structural characterization revealed that the bioflocculant was mainly composed of 37.50% neutral sugar, 10.03% uronic acid, 6.32% aminosugar and 16.51% protein. Carboxyl, amine and hydroxyl groups were the functional groups in flocculation. The biofocculant was thermally stable and dependent on metal ions and acidic pH, showing a good flocculating activity of 91.92% at the dosage of 25 mg/L by aid of 1.0 mM Fe3+ at pH 2.0. Due to these unique properties, the bioflocculant could efficiently remove metal ions such as Fe, Al, Zn, and Pb from the real acid mine wastewater sample without pH adjustment, and meanwhile made the acid mine wastewater solution become clear with an increased neutral pH. These findings suggested the great potential application of the non-toxic bioflocculant from C. glutamicum Cg1-P30 in acid mine wastewater treatment.
Introduction
The removal of colloidal particles mediated by flocculants is an important procedure in many industrial fields such as tap water purification and wastewater treatment, dyes and textiles, food and beverage. Flocculants are generally divided into three different categories: inorganic, organic, and bioflocculants (Alias et al., 2022). Compared with conventional chemical inorganic and organic flocculants, microbial bioflocculants, the macromolecules secreted by microorganisms during growth and lysis, are considered to be ecosystem-friendly due to their non-toxic and biodegradable properties. In addition, microbial bioflocculants can be produced at high rates and the extracellular bioflocculants are easily recovered from the fermentation broth. Thus, microbial biofluccolants are attracting extensive attention as a potential alternative to chemical flocculants (Shahadat et al., 2017).
A number of microorganisms, including bacteria, fungi, microalgae and actinomycetes, have been reported to produce bioflocculants. For example, Bacillus sp. is the most studied bacterial species for bioflocculant production (Bakar et al., 2021). However, the practical application of bioflocculants is still limited due to their low flocculating efficiency, low yield, and high cost of production. Consequently, it become important to screen novel effective bioflocculant-producing microorganisms, seek for economical cultivation substrates and optimize the fermentation process in the research of bioflocculant (Okaiyeto et al., 2016). At the same time, the performance of biological flocculants is closely related to its structural composition (Kurniawan et al., 2022), and is greatly affected by flocculation conditions such as dosage, metal ions, pH value, and temperature. Therefore, it is beneficial to master this information for selecting suitable bioflocculants facing with application scenarios with different characteristics. Corynebacterium glutamicum is generally recognized as safe (GRAS) and have been widely used as host for production of functional compounds, however, to date there have been few reports on production, characterization, properties of biofluccolants from Corynebacterium glutamicum, except a polygalacturonic acid bioflocculant named REA-11 from C. glutamicum CCTCC M201005 (He et al., 2002; Li et al., 2003; He et al., 2004a; He et al., 2004b).
Acid mine wastewater, which is an unavoidable by-product of the mining and mineral industry, is harmful to the aquatic environment because it contains high concentrations of dissolved heavy metals (primarily iron, aluminum, zinc, and other heavy metals) and sulphate, and have a high turbidity and a high acidity with a pH of 2.0–3.0 (Feng et al., 2004; Kefeni et al., 2017; Fu et al., 2020). Acid mine wastewater has become the leading environmental issue of the mining industry over the years, many methods, containing alkaline chemical neutralization, precipitation, adsorption, and membrane technology have been widely used in treatment of acid mine wastewater. However, though the utilization of biolflocculant is thought as an economic and effective strategy to treat the mining wastewater, such as clarification of turbid aqueous solutions (Liu et al., 2019), removal of organics (Maliehe et al., 2019) and metal remediation (Ayangbenro et al., 2019), probably because the flocculating activities of many reported bioflocculants have been significantly inhibited in high acidic conditions or in the presence of iron or aluminum (Tawila et al., 2019; Pu et al., 2020; Rajivgandhi et al., 2021), the application of bioflocculant in acid mine wastewater treatment is still need to be explored.
In this study, a novel bioflocculant was produced from C. glutamicum Cg1-P30, which was Gram-positive, catalase positive with rod-like shapes and identified by 16S rRNA sequence analysis (Supplementary Material), and the culture medium using low-cost corn steep liquor as a nutrient source for fermentation was optimized. The structural characterization, flocculating activities, and the application in treatment of acid mine wastewater of the isolated bioflocculant were also investigated.
Materials and methods
Culture conditions
Corynebacterium glutamicum Cg1-P30 (CMCC NO. 22680), preserved at China General Microbiological Culture Collection Center, was initially grown in brain heart infusion (BHI) agar medium for activation. The activated strain was maintained on BHI broth at 30°C in a rotary shaker at 200 rpm overnight. Subsequently, 2% of the seed culture was transferred into new flasks containing 50 mL of production medium consisting of 10 g/L glucose, 5 g/L corn steep liquor, 5 g/L urea, 0.25 g/L MgSO4·7 H2O, 1.0 g/L K2HPO4 and 1.0 g/L KH2PO4 and incubated at 30°C and 200 rpm for 48 h. The concentrations of glucose, corn steep liquor, and urea were further optimized as described in the section of optimization of medium for bioflocculant production.
Optimization of medium for bioflocculant production
Based on the result of one-factor-at-a-time experiment, response surface methodology through Box-Behnken design (Design Expert software, Version 8.0.6) was applied to evaluate the optimum level and interactive effects of carbon and nitrogen sources on bioflocculant production. The concentration of glucose, corn steep liquor, and urea were selected as independent variables at three different levels, while the response variable was the flocculating activity of the fermentation supernatant after culturing for 48 h. The result of Box-Behnken design was shown in Table 1. A quadratic polynomial equation was fitted to evaluate the correlate relationship between the independent variables and the response as following:
Where Y (%) is the flocculating activity of the fermentation supernatant, β0 is the intercept coefficient; β1, β2, and β3 are the linear coefficients; β11, β22, and β33 are the quadratic coefficients; and β12, β13, and β23 are the interaction coefficients; X1, X2, X3 are the concentration of glucose, corn steep liquor, and urea, respectively. Three additional experiments were conducted to verify the validity of the statistical experimental strategies.
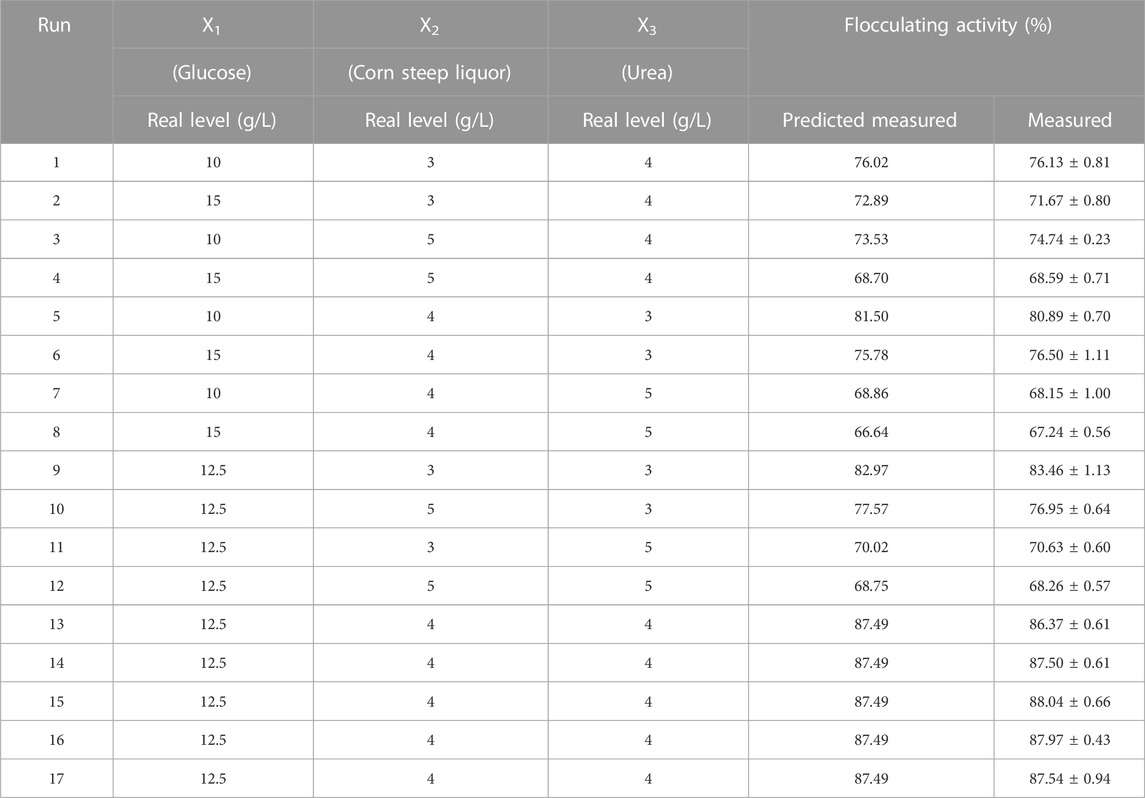
TABLE 1. The matrix of the BBD experiment for culture medium optimization and the experimental and predicted flocculating activity.
Determination of flocculating activity
The flocculating activity was determined using the kaolin clay suspension method (Sun et al., 2012) with some modification. 1 mL bioflocculant sample (the fermentation supernatant was used in the optimization experiment) and 50 μL FeCl3 (1 M) solution were mixed with 49 mL of kaolin solution (4 g/L) and then incubated for 5 min at room temperature. The absorbance of the upper phase of the mixture was measured by at 550 nm. A control in which the bioflocculant was replaced with deionized water was also conducted under the same conditions. The flocculating activity was calculated according to the following equation:
Where A and B are the absorbance at 550 nm of the sample and control, respectively.
Extraction and purification of bioflocculant
The cell-free supernatant obtained from the culture broth by centrifugation at 6,000 rpm and 4°C for 30 min was mixed with three volumes of ethanol and kept at 4°C overnight for precipitation. The resulting precipitate by centrifugation was dissolved in distilled water to repeat the precipitation procedure for three times. The final collected precipitate was dissolved in deionized water, dialyzed (molecular weight cutoff of 10,000 Da) to remove low molecular weight compounds and lyophilized at −50°C to obtain the purified bioflocculant.
Characterization of the bioflocculant
The total content of polysaccharide, uronic acid, amino sugar and protein in the bioflocculant was measured by the phenol-sulfuric acid method, carbazole-sulfate method, Elson-Morgan method and Bradford method, with glucose, glucuronic acid, glucosamine and bovine albumin as the standard, respectively. For monosaccharide composition analysis, after hydrolysis of the bioflocculant with trifluoroacetic acid at 110°C for 6 h, the hydrolyzed sample was analyzed by using a high-pressure ion chromatography system (Dionex ICS 3000, Thermo Fisher Scientific Inc., United States), equipped with an anion exchange column (Dionex CarboPac PA20, Thermo Fisher Scientific Inc., United States) and a pulsed amperometric detector (reference electrode Ag-AgCl, measuring electrode Au). For neutral monosaccharide analysis, the mobile phase consisting of A (100 mM NaOH) and B (10 mM NaOH), was programmed as follows: 0–5 min, 100% A; 5–25 min, 10% A. For uronic acid monosaccharide analysis, the mobile phase consisting of A (10 mM NaOH) and B (100 mM NaOH containing 1 M sodium acetate), was programmed as follows: 0–20 min, 95% A; 20–30 min, 70% A; 30–35 min, 100% A. The identification of each monosaccharide peak was determined on the basis of the elution time by comparison with standard solutions of different monosaccharides (fucose, arabinose, rhamnose, galactose, glcosamine, glcose, xylose, galacturonic acid and glucuronic acid). The FT-IR spectra of the bioflocculant was recorded after grinded with KBr powder and pressed into a pellet, by a Bruker Vertex 70 spectrometer (Bruker Optics, United States) at the wavelength range of 400–4,000 cm−1. The elemental composition of the bioflocculant was analyzed using a Thermo Scientific K-Alpha XPS system (Thermo Fisher Scientific Inc., United States). The surface morphology of the bioflocculant was observed by SEM (Hitachi SU8010, Japan).
Measurement of the flocculating activity
The influence of pH was tested in the kaolin clay suspension system with a pH of 2.0–8.0 adjusted by 2 M HCl or 2 M NaOH. The cations including NaCl, KCl, MgCl2, CaCl2, CuCl2, FeCl2, FeCl3, and AlCl3 were used to test the effect of metal ions on the flocculating activity at the final concentration of 1.00 mM. The dose effects of the biopolymer (5–30 mg/L) and Fe3+ (0.50–1.50 mM) were studied at pH 2.0. The thermal stability was test at the temperature of 20, 40, 60, 80, and 100°C. The zeta potential of kaolin suspension before and after flocculation was analyzed by a Zetasizer (Zetasizer Pro, Malvern, United Kingdom).
Application in acid mine wastewater
The acid mine wastewater was sampled from iron ore processing plant of Xiangtan City in Hunan Province, China. In order to analyze the application potential of the bioflocculant in acid mine waste water treatment, the biofluccolant was added into the acid mine wastewater at the final concentration of 25 mg/L without pH adjustment. The suspension was shaken at 200 rpm and 30°C for 30 min. Then the flocculating activity was calculated according to the change of turbidity based on the absorbance at 550 nm and the pH change was record by a pH meter ((FiveEasy Plus pH, Mettler, United States). The supernatant was collected for detecting the concentration of Fe, Al, Mn, Cu, Pb, Zn, Ni and Cd by inductively coupled plasma atomic emission spectroscopy (ICP-AES, Hitachi Limited., Japan). The metal removal rate was calculated as follows:
where C0 and C were the initial and final concentrations of the metal, respectively.
Statistical analysis
Data are expressed as mean values with standard deviation (±SD) from three independent experiments. Statistical comparisons were performed using analysis of variance (ANOVA), and differences at p < 0.05 were considered statistically significant.
Result
Optimization of fermentation medium composition
A three-level, three-factor Box-Behnken design was used to optimize the medium composition containing corn steep liquor in shake-flask fermentation. The obtained quadratic regression model representing the flocculating activity as a function of the carbon and nitrogen source was written as follows:
where Y was the flocculating activity of the fermentation supernatant, X1, X2, X3 were the concentration of glucose, corn steep liquor, and urea, respectively. The statistical significance of the regression model was assessed by F-test and p-value. The analysis of variance (ANOVA) for the quadratic model was summarized in Table 2. Similarly to the study of Agunbiade et al. (2022), the model F-value of 99.09 indicated that the model was highly statistically significant at p < 0.0001. The lack of fit F-value and p-value was found to be 4.4 and 0.093, indicating that the suitability of the model to predict the variations. The p-values showed that X1, X2, X3, X12, X22, and X32 were statistically significant at the 95% confidence level. In contrast, X1X2, X1X3, and X2X3 had no significant influence on the flocculating activity. In addition, the value of the determination coefficient (R2), the adjusted determination coefficient (R2adj) and the predicted determination coefficient determination coefficient (R2pred) were 0.9922, 0.9811, and 0.9015, respectively, indicating a good correlation between experimental and theoretical results. At the same time, a low value (1.36%) of the coefficient of the variation (C.V.) demonstrated the experimental values were precise and reliable.
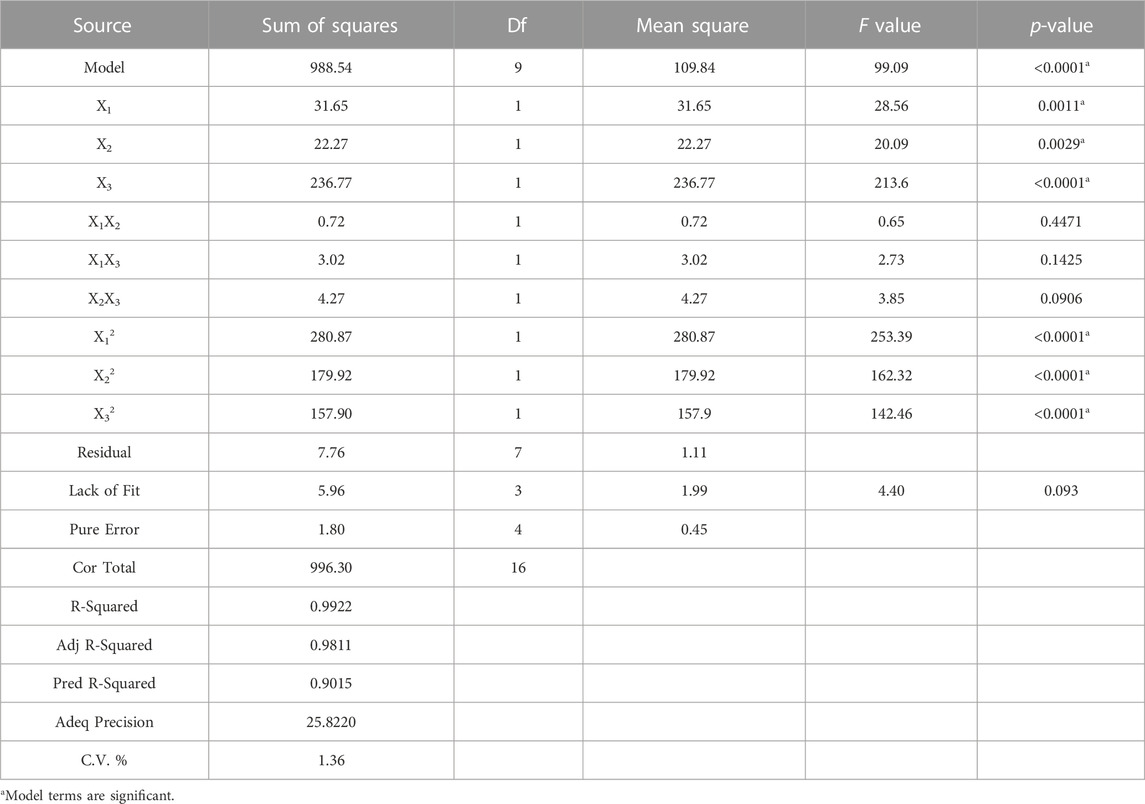
TABLE 2. Variance analysis (ANOVA) of the response surface quadratic model for culture medium optimizaition.
From the regression model analysis, a maximum flocculating activity of the fermentation broth was estimated as 88.93% under the optimal medium composition with glucose at 12.20 g/L, corn steep liquor at 4.00 g/L and urea at 3.60 g/L. Under this optimal condition, the actual flocculating activity was 88.79% and was very close to the predicted value. By combination of repeated precipitation and dialysis, 0.52 g of purified bioflocculant was finally obtained from 1 L of fermentation broth.
Characterization of the bioflocculant
Chemical analysis revealed that the purified bioflocculant contained neutral sugar (37.50%), uronic acid (10.03%), aminosugar (6.32%), protein (16.51%) and nucleic acid (3.72%). The detected rhamnose, arabinose, galactose, glucose, mannose, fucose, galacturonic acid and glucuronic acid in monosaccharide composition analysis were at a molar ratio of 1.11:2.29:1.84:3.67:4.67:2.93:5.67:4.85 (Figure 1).
The FTIR spectrum of the purified bioflocculant was displayed in Figure 2. The broad band around 3,420 cm−1 was representative of the stretching vibration of O-H group. The weak band at 2,930 cm−1 was attributed to the stretching vibration of C-H aliphatic bands (Huang et al., 2019). The band at 1,640 cm−1 was resulted from C=O stretching vibration (Poorgholy et al., 2017). The bands at 1,557 and 1,407 cm−1 were generated by N-H and C-H bending vibration, respectively (Salaberria et al., 2014; Yang et al., 2022) The band around 1,059 cm−1 was from the stretching vibration of the C-O group (Fang et al., 2021).
The elemental composition of the biofocculant was analyzed by XPS. The mass fraction of C, O, N, S, and P was detected as 63.48%, 30.58%, 4.65%, 1.00%, and 0.29%, respectively. Moreover, the core level peaks of C 1s, O 1s and N 1s were deconvoluted to vertify the corresponding functional groups. As Figure 3 shown, the peaks located at 284.7, 286.2, and 287.6 eV in the C 1s high-resolution spectrum were assigned to C–C, C–OH/C–N and C=O groups, respectively (Li et al., 2009). The peaks located at 531.5, 532.3, and 533.1 eV in the O 1s high-resolution spectrum were attributed to C=O, O–C–O/O-C=O and C-OH, respectively (Liang et al., 2020). The peak at 400.28 eV in the N 1s high-resolution spectrum was assigned to the protonated nitrogen in aminosugar, while the peak at 399.5 eV was originated from the non-protonated nitrogen in NH or NH2 group (Zhang et al., 2013; Zhong et al., 2018).
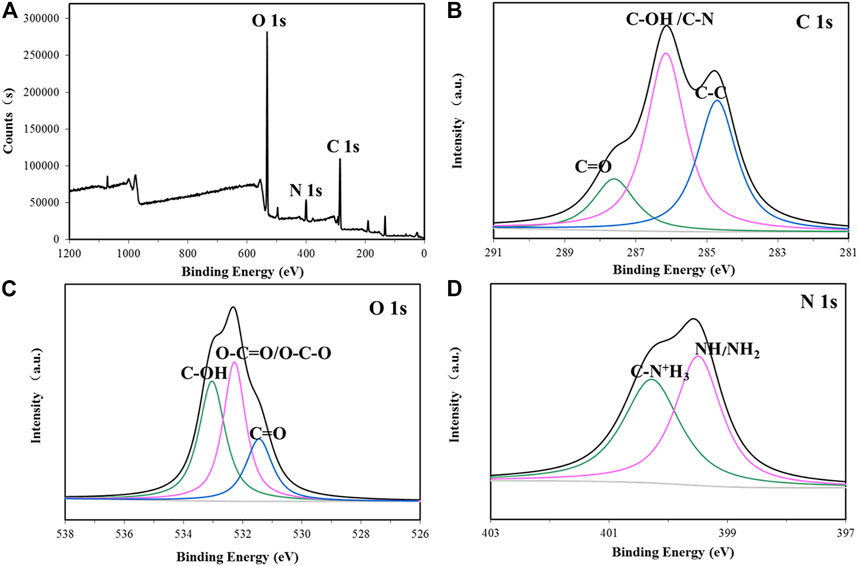
FIGURE 3. XPS spectra of the bioflocculant (A) and high resolution 1 s XPS spectra of C, O, and N from P-GS408 are shown in (B–D), respectively.
Measurement of the flocculating activity
Effects of metal ions, pH, dosage, and temperature on flocculating activity of the bioflocculant were shown in Figure 4. The bioflocculant alone had negligible flocculating activity, but the situation changed by addition of metal ions. At pH 2.0, the bioflocculant at 10 mg/L demonstrated a good flocculating activity of 88.79% and 82.72% toward 4 g/L kaolin suspension, in the presence of 1.0 mM FeCl3 and AlCl3, respectively. If the metal ion changed or the pH increased, the flocculating activity dramatically dropped. The trivalent cations were more favorable for promoting flocculation than divalent and monovalent cations.
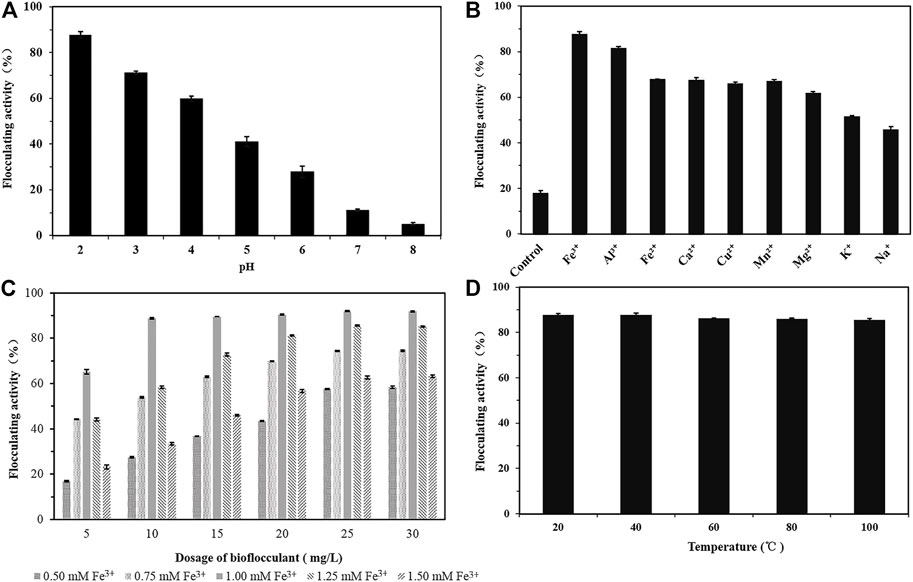
FIGURE 4. The effects of pH (A), cations (B), dosage of the bioflocculant and Fe3+ (C), temperature (D) on the flocculating activity of Kaolin suspension by the bioflocculant.
Considering that though the zeta potential of kaolin solution at pH 2.0 was changed from −12.8 to 9.55 mV by addition of the bioflocculant, no obvious fluccolation was observed, however, in the presence of both Fe3+ and the bioflocculant, the zeta potential of kaolin solution changed to 35.45 mV and the flocculation efficiency was significantly improved, it was speculated that trivalent cations with higher charge density could more effectively neutralize the negative charge of kaolin particles to form bridges (Salehizadeh et al., 2000). Variations in pH also cause differences in the electrostatic charge of the suspended particles, thus affecting the bridging efficiency for kaolin clay particles. The absolute values of zeta potential of kaolin suspension in the study were increased from −12.8 to −39.9 mV with the increase of pH 2.0 to 6.0.
The synergistic effect of bioflocculant and Fe3+ on the flocculating activity was tested at different dosages of bioflocculant and FeCl3. The maximum flocculating activity achieved 91.92% at the dosage of 25 mg/L biolfocculant by combination of 1.0 mM Fe3+. When the bioflocculant concentration varied from 10 mg/mL to 30 mg/mL, its flocculating activity kept increasing with the increase of Fe3+ concentration from 0.5 to 1.0 mM, and at the presence of 1.0 mM Fe3+ the flocculating activities were above 88% with not much change during this bioflocculant concentration range, but overdosage of Fe3+ would reverse the surface charge of kaolin particles from negative to positive and reduced flocculation obviously by electrostatic repulsion forces (Wang et al., 2015; Nguyen et al., 2021; Hyrycz et al., 2022). With respect to the influence of temperature, the bioflocculant retained high flocculating activity above 85% when temperature varied from 20°C to 100°C, indicated it was stable under high temperature.
Application in acid mine wastewater treatment
A real acid mine wastewater sample without pH adjustment was directly used to investigate the application possibility of the bioflocculant in treatment of acid mine wastewater. As shown in Figure 5, after treatment with the bioflocculant at a dosage of 25 mg/L for 30 min, the acid mine wastewater sample changed from yellow-brown to colorless and clarified, meanwhile, an amount of yellow-brown sediment appeared at the bottom, and the flocculating activity was tested as 96.39%. The yellow-brown color of the acid mine wastewater was caused by large quantities of ferric ions resulted from the oxidation of ferrous ions, and ferric ions could start to precipitate at a pH as low as 3.0 (Potgieter-Vermaak et al., 2006), it seemed that the addition of the bioflocculant accelerated the precipitation process of ferric ions by flocculation. ICP analysis further confirmed that the bioflocculant was able to remove metal ions from the acid mine wastewater sample, with a removal rate higher than 70% to Fe, Al, Zn, Pb, and Cd. Under the action of the bioflocculant, the final concentration of Pb2+ was lower than 1 mg/L, meanwhile, though the final concentration of Mn2+ and Cu2+ did not meet this standard, their concentration obviously changed from 49.7 to 28.6 mg/L and 5.72 to 2.45 mg/mL, respectively. Moreover, the pH of the acid mine wastewater was effectively increased from 3.04 to 6.86. The zeta potential of the acid mine wastewater was changed from −19.12 to 2.89 mV by addition of the bioflocculant. SEM images showed that the bioflocculant had an irregular stacked layer structure and aggregated with acid mine wastewater to form large clumps with net-like structure (Figure 6).
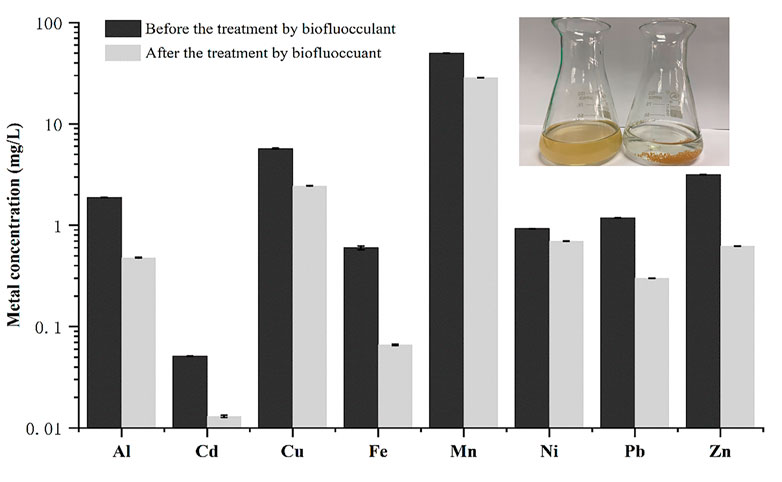
FIGURE 5. The effect of the bioflocculant on the metal ion concentration of the real acid wine wastewater.
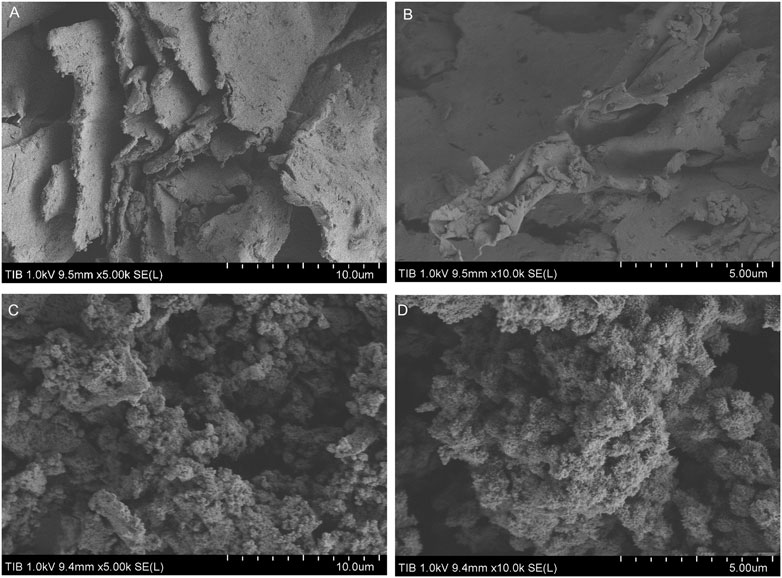
FIGURE 6. SEM micrograph images of purified bioflocculant (A,B) and bioflocculant aggregation with the acid mine wastewater (C,D).
Discussion
Fermentation medium accounts for a significant portion in the overall cost of bioflocculant production (Pu et al., 2018; Fan et al., 2019; Mohammed and Dagang, 2019) especially carbon and nitrogen source, plays a vital role (Xia et al., 2018b). Corn steep liquor, the by-product of corn wet-milling, has been used as cheap nutrient supply for the fermentation of various microorganisms, due to its high contents of soluble proteins, amino acids, carbohydrates, vitamins and minerals (de Barros et al., 2020; Kang et al., 2020). The bioflocculant fermentation production process using corn steep liquor as a cheap nutrient supply was successfully accomplished by response surface methodology in this study. According to the p-values, the corn steep liquor, glucose and urea all showed significant impact on the yield of bioflocculant production, however, the interaction between glucose, corn steep liquor, and urea was not significant. Though fed-batch cultivation strategy and two-stage pH control mode had been applied to improve the production of bioflocculant REA-11 from C. glutamicum CCTCC M201005 (He et al., 2004b; Wu et al., 2010), its reported yield was only calculated in U/mL, thus it was difficult to compare the result with the yield of bioflocculant in this study using the common unit g/L. Meanwhile, considering that only 0.24, 0.26, 0.40, and 0.36 g of purified bioflocculant was obtained from 1 L of fermentation broth of Klebsiella oxytoca GS-4-08 (Yu et al., 2016), Virgibacillus sp. Rob (Cosa et al., 2011), Aspergillus flavus S44-1 (Aljuboori et al., 2013) and Bacillus amyloliquefaciens DT (Sun et al., 2015), respectively, the bioflocculant production yield of 0.52 g/L in this study though was not high, but was still acceptable. Besides that C. glutamicum was not an efficient bioflocculant producer, it was speculated that the low production yield might be attributed to that only the culture medium composition was partially optimized, other fermentation conditions, such as pH, time and inoculum size, should be optimized in further study.
The monosaccharide composition of the bioflocculant in this study was very different from that of the bioflocculant REA-11 produced by C. glutamicum CCTCC M201005, which was composed of galacturonic acid with trifle proteins (He et al., 2002), but had some similarities to many microbial bioflocculants (Sun et al., 2015; Chen et al., 2017; Xia et al., 2018a; Xia et al., 2022). The polysaccharide was the main ingredient in the bioflocculant and the carboxyl groups of uronic acid in the bioflocculant could provide a certain amount of effective sites for the attachment of particles (Xia et al., 2022). The FTIR spectrum showed the presence of carboxyl, hydroxyl, and amino groups in the bioflocculant, and the result of XPS was in agreement with FTIR analysis, further confirmed that the hydroxyl, carboxyl and amino groups were abundant in the bioflocculant (Fan et al., 2019).
The bioflocculant REA-11 originated from C. glutamicum CCTCC M201005 was stable at pH 3.0–6.5 and its flocculating activity was markedly improved by Ca2+. Under the optimum bioflocculant concentration of 8.2 mg/L and optimum CaCl2 concentration of 8.0 mM, its flocculating activity was 85.2% (He et al., 2004a). However, the flocculating activity of the biofloccuant in this study was different from that of REA-11, its enhanced flocculating activity induced by FeCl3 or AlCl3 was similar to that of the bioflocculants produced by Bacillus sp. As-101 (Salehizadeh et al., 2000), K. oxytoca GS-4-08 (Yu et al., 2016), Raoultella ornithinolytica 160–1 (Ding et al., 2021), Klebsiellu sp. A9 (Sheng et al., 2016) and Halogeometricum borinquense A52 (Chouchane et al., 2020). Moreover, the maximum flocculating activity of the bioflocculant observed at pH 2.0 was similar to that of the bioflocculants produced by Bacillus sp. F19 (Zheng et al., 2008), Bacillus aryabhattai PSK1 (Abd El-Salam et al., 2017) and Azotobacter chroococcum (Yang et al., 2017). Kaolin clay particles form an electrical double layer at the solid-water interfaces and the negative charge on the surface of the particles enables them to suspend well in the solution. According to Elkady et al. (2011), the good flocculating activity at pH 2.0 might be attributed to that the electric double layer was changed because the negative charge on the surface was reduced by the adsorption of H+ in the highly acidic environment, subsequently the electrostatic repulsion force and distance between the suspended particles were decreased, and the bridging effect of the bioflocculant was correspondingly improved. The optimal flocculation conditions of the bioflocculant from Bacillus sp. for kaolin solution were determined as 120 mg/L bioflocculant and 0.4 mM Fe3+ (Hua et al., 2021). As for the bioflocculant from K. oxytoca GS-4-08, the best dosage of the bioflocculant and Fe3+ were 700 mg/L and 3.0 mM respectively in the flocculating system (Yu et al., 2016). Compared to these reported bioflocculants, the bioflocculant in this study achieved a high flocculating activity at a relative low dosage by the aid of Fe3+. Chouchane et al. (2018) reported that the heteropolysaccharide-based bioflocculant from hydrocarbonoclastic strain Kocuria rosea BU22S was thermally stable. Pu et al. (2020) found that the polysaccharide BM2 produced by Bacillus megaterium strain PL8 had a flocculating activity over 87% from 20°C to 100°C. The thermostability of the bioflocculant in this study is in accordance with these reports. Moreover, considering that biofluccolants with polysaccharide backbone were thermostable, while those with high compositions of proteins were generally sensitive to heat (Tawila et al., 2018), the good thermo-stability of the bioflocculant indicated that its flocculating activity was primarily resulted from the polysaccharide-based structure, rather than protein.
Acid mine wastewater has a low pH of 2.0–3.0 and contains high levels of different metals such as iron, aluminium, manganese, zinc, lead, copper and nickel (Vital et al., 2018). The high acid value and the existence of the metal ions in acid mine wastewater are both beneficial to the performance of the bioflocculant in this study. The flocculation behavior of the bioflocculant in the real acid mine wastewater sample illustrated that some metal ions underwent co-precipitation with ferric ions and was removed from the wastewater by the help of the bioflocculant. Meanwhile, due to the precipitation of sulfides, hydroxides, and carbonate (Chen et al., 2021), the pH of the wastewater changed from acid to close to neutral. After treatment by the bioflocculant, the final concentration of Pb2+ and the pH in the wastewater sample reached the national wastewater standard stipulated in the Integrated Wastewater Discharge Standard (Yan et al., 2015). The zeta potential change of the acid mine wastewater with the addition of the bioflocculant showed that the charge neutralization mechanism was involved in the flocculation. Moreover, the interstitial spaces between the stacked layers of the bioflocculant observed by SEM were beneficial to the absorption of metal ions (Saba et al., 2019), while the net-like structure of the flocs after flocculation indicated that polymer bridging played an important role in the flocculation process (Aljuboori et al., 2015). Considering that the mineral content and concentration of water-soluble metal ions in the acid mine water varies with the geological environment in different mining areas, in the further study, the action of the bioflocculant needs to be tested using more different acid mine water samples, at the same time, its application condition requires more detailed exploration.
In conclusion, a novel cation-dependent bioflocculant was produced by the GRAS strain C. glutamicum utilizing corn steep liquor as a cheap nutrient supply. Chemical analysis indicated that the bioflocculant was mainly composed of polysaccharides (53.85%) and proteins (16.51%). FTIR and XPS analysis revealed the abundant carboxyl, amine and hydroxyl groups in its structure. The bioflocculant had high thermal stability, and showed a good flocculating activity over 70% to kaolin clay suspension by the aid of Fe3+ at high acidic condition. Moreover, the simple addition of bioflocculant to the real acid mine wastewater could remove metal ions such as Fe, Al, Pb, Zn efficiently from the sample, making the suspension clear with an increase in the pH to neutral. These findings indicated the bioflocculant from C. glutamicum could be an efficient and promising material to treat acid mine wastewater in a simple and fast way.
Data availability statement
The raw data supporting the conclusions of this article will be made available by the authors, without undue reservation.
Author contributions
YL conducted experiments and analyzed data. YZ analyzed data and wrote the manuscript. JY and PC conducted experiments. YS and MW revised the manuscript. YM conceived and designed research and revised the manuscript. All authors read and approved the manuscript.
Funding
This work was supported by National Key R&D Program of China (No. 2019YFA0904900).
Conflict of interest
The authors declare that the research was conducted in the absence of any commercial or financial relationships that could be construed as a potential conflict of interest.
Publisher’s note
All claims expressed in this article are solely those of the authors and do not necessarily represent those of their affiliated organizations, or those of the publisher, the editors and the reviewers. Any product that may be evaluated in this article, or claim that may be made by its manufacturer, is not guaranteed or endorsed by the publisher.
Supplementary material
The Supplementary Material for this article can be found online at: https://www.frontiersin.org/articles/10.3389/fbioe.2023.1136473/full#supplementary-material
Supplementary Figure S1 | The 16S rRNA gene sequence of C. glutamicum Cg1-P30.
Supplementary Figure S2 | Neighborhood joining tree of C. glutamicum Cg1-P30.
Supplementary Figure S3 | SEM micrograph images of purified bioflocculant (A), Kaolin (B) and bioflocculant aggregation with Kaolin (C).
References
Abd El-Salam, A. E., Abd-El-Haleem, D., Youssef, A. S., Zaki, S., Abu-Elreesh, G., and El-Assar, S. A. (2017). Isolation, characterization, optimization, immobilization and batch fermentation of bioflocculant produced by Bacillus aryabhattai strain PSK1. J. Genet. Eng. Biotechnol. 15 (2), 335–344. doi:10.1016/j.jgeb.2017.07.002
Agunbiade, M., Oladipo, B., Ademakinwa, A. N., Awolusi, O., Adesiyan, I. M., Oyekola, O., et al. (2022). Bioflocculant produced by Bacillus velezensis and its potential application in brewery wastewater treatment. Sci. Rep. 12, 10945. doi:10.1038/s41598-022-15193-8
Alias, J., Hasan, H. A., Abdullah, S. R. S., and Othman, A. R. (2022). Properties of bioflocculant-producing bacteria for high flocculating activity efficiency. Environ. Technol. Inno. 27, 102529. doi:10.1016/j.eti.2022.102529
Aljuboori, A. H. R., Idris, A., Abdullah, N., and Mohamad, R. (2013). Production and characterization of a bioflocculant produced by Aspergillus flavus. Bioresour. Technol. 127, 489–493. doi:10.1016/j.biortech.2012.09.016
Aljuboori, A. H. R., Idris, A., Al-Joubory, H. H. R., Uemura, Y., and Abubakar, I. B. S. U. (2015). Flocculation behavior and mechanism of bioflocculant produced by Aspergillus flavus. J. Environ. manage. 150, 466–471. doi:10.1016/j.jenvman.2014.12.035
Ayangbenro, A. S., Babalola, O. O., and Aremu, O. S. (2019). Bioflocculant production and heavy metal sorption by metal resistant bacterial isolates from gold mining soil. Chemosphere 231, 113–120. doi:10.1016/j.chemosphere.2019.05.092
Bakar, S. N. H. A. H., Hasan, A., Abdullah, S. R. S., Kasan, N. A., Muhamad, M. H., and Kurniawan, S. B. (2021). A review of the production process of bacteria-based polymeric flocculants. J. Water Process Eng. 40, 101915. doi:10.1016/j.jwpe.2021.101915
Chen, G., Ye, Y., Yao, N., Hu, N., Zhang, J., and Huang, Y. (2021). A critical review of prevention, treatment, reuse, and resource recovery from acid mine drainage. J. Clean. Prod. 329, 129666. doi:10.1016/j.jclepro.2021.129666
Chen, Z., Li, Z., Liu, P., Liu, Y., Wang, Y., Li, Q., et al. (2017). Characterization of a novel bioflocculant from a marine bacterium and its application in dye wastewater treatment. BMC Biotechnol. 17, 84. doi:10.1186/s12896-017-0404-z
Chouchane, H., Mahjoubi, M., Ettoumi, B., Neifar, M., and Cherif, A. (2018). A novel thermally stable heteropolysaccharide-based bioflocculant from hydrocarbonoclastic strain Kocuria rosea BU22S and its application in dye removal. Environ. Technol. 39 (7), 859–872. doi:10.1080/09593330.2017.1313886
Chouchane, H., Najjari, A., Neifar, M., Cherif, H., Askri, R., Naili, F., et al. (2020). Unravelling the characteristics of a heteropolysaccharide-protein from an Haloarchaeal strain with flocculation effectiveness in heavy metals and dyes removal. Environ. Technol. 41 (17), 2180–2195. doi:10.1080/09593330.2018.1556742
Cosa, S., Mabinya, L. V., Olaniran, A. O., Okoh, O. O., Bernard, K., Deyzel, S., et al. (2011). Bioflocculant production by Virgibacillus sp. rob isolated from the bottom sediment of algoa bay in the eastern cape, south Africa. Molecules 16 (3), 2431–2442. doi:10.3390/molecules16032431
de Barros, P. D. S., e Silva, P. E. C., Nascimento, T. P., Costa, R. M. P. B., Bezerra, R. P., and Porto, A. L. F. (2020). Fibrinolytic enzyme from Arthrospira platensis cultivated in medium culture supplemented with corn steep liquor. Int. J. Biol. Macromol. 164, 3446–3453. doi:10.1016/j.ijbiomac.2020.08.217
Ding, R., Luo, L., Han, R., Zhang, M., Li, T., Tang, J., et al. (2021). Rapid production of a novel Al (III) dependent bioflocculant isolated from Raoultella ornithinolytica 160-1 and its application combined with inorganicsalts. Front. Microbiol. 11, 622365. doi:10.3389/fmicb.2020.622365
Elkady, M. F., Farag, S., Zaki, S., Abu-Elreesh, G., and Abd-Ei-Haleem, D. (2011). Bacillus mojavensis strain 32A, a bioflocculant-producing bacterium isolated from an Egyptian salt production pond. Bioresour. Technol. 102, 8143–8151. doi:10.1016/j.biortech.2011.05.090
Fan, H., Yu, J., Chen, R., and Yu, L. (2019). Preparation of a bioflocculant by using acetonitrile as sole nitrogen source and its application in heavy metals removal. J. Hazard Mat. 363, 242–247. doi:10.1016/j.jhazmat.2018.09.063
Fang, K., Wang, B., Zhang, Y., and Li, H. (2021). Optimized production and characterization of cation-independent bioflocculant produced by Klebsiella sp. 59L. Environ. Sci. Pollut. Res. Int. 28, 7981–7993. doi:10.1007/s11356-020-11162-8
Feng, D., van Deventer, J. S. J., and Aldrich, C. (2004). Removal of pollutants from acid mine wastewater using metallurgical by-product slags. Sep. Purif. Technol. 40 (1), 61–67. doi:10.1016/j.seppur.2004.01.003
Fu, W., Ji, G., Chen, H., Yang, S., Guo, B., Yang, H., et al. (2020). Molybdenum sulphide modified chelating resin for toxic metal adsorption from acid mine wastewater. Sep. Purif. Technol. 251 (4), 117407. doi:10.1016/j.seppur.2020.117407
He, N., Li, Y., Chen, J., and Lun, S. Y. (2002). Identification of a novel bioflocculant from a newly isolated Corynebacterium glutamicum. Biochem. Eng. J. 11, 137–148. doi:10.1016/S1369-703X(02)00018-9
He, N., Li, Y., and Chen, J. (2004a). Production of a novel polygalacturonic acid bioflocculant REA-11 by Corynebacterium glutamicum. Bioresour. Technol. 94, 99–105. doi:10.1016/j.biortech.2003.11.013
He, N., Wu, X., Deng, X., Lu, Y., and Li, Q. (2004b). Two-stage pH control mode in batch fermentation of a novel bioflocculant from Corynebacterium glutamicum. Chem. Res. Chin. Univ. 20 (2), 152–155.
Hua, J., Zhang, R., Chen, R., Liu, G., Yin, K., and Yu, L. (2021). Energy-saving preparation of a bioflocculant under high-salt condition by using strain Bacillus sp. and the interaction mechanism towards heavy metals. Chemosphere 267, 129324. doi:10.1016/j.chemosphere.2020.129324
Huang, J., Huang, Z. L., Zhou, J. X., Li, C. Z., Yang, Z. H., Ruan, M., et al. (2019). Enhancement of heavy metals removal by microbial flocculant produced by Paenibacillus polymyxa combined with an insufficient hydroxide precipitation. Chem. Eng. J. 374, 880–894. doi:10.1016/j.cej.2019.06.009
Hyrycz, M., Ochowiak, M., Krupińska, A., Włodarczak, S., and Matuszak, M. (2022). A review of flocculants as an efficient method for increasing the efficiency of municipal sludge dewatering: Mechanisms, performances, influencing factors and perspectives. Sci. Total Environ. 820, 153328. doi:10.1016/j.scitotenv.2022.153328
Kang, C. K., Jeong, S. W., Yang, J. E., and Choi, Y. J. (2020). High-yield production of lycopene from corn steep liquor and glycerol using the metabolically engineered Deinococcus radiodurans R1 Strain. J. Agri.c Food Chem. 68 (18), 5147–5153. doi:10.1021/acs.jafc.0c01024
Kefeni, K. K., Msagati, T. A. M., and Mamba, B. B. (2017). Acid mine drainage: Prevention, treatment options, and resource recovery: A review. J. Clean. Prod. 151, 475–493. doi:10.1016/j.jclepro.2017.03.082
Kurniawan, S. B., Imron, M. F., Chik, C. E. N. C. E., Owodunni, A. A., Ahmad, A., et al. (2022). What compound inside biocoagulants/bioflocculants is contributing the most to the coagulation and flocculation processes? Sci. Total Environ. 806, 150902. doi:10.1016/j.scitotenv.2021.150902
Li, Y., He, N., Guan, H., Du, G., and Chen, J. (2003). A novel polygalacturonic acid bioflocculant REA-11 produced by Corynebacterium glutamicum: A proposed biosynthetic pathway and experimental confirmation. Appl. Microbiol. Biotechnol. 63 (2), 200–206. doi:10.1007/s00253-003-1365-9
Li, Z., Zhong, S., Lei, H., Chen, R., Yu, Q., and Li, H. L. (2009). Production of a novel bioflocculant by Bacillus licheniformis X14 and its application to low temperature drinking water treatment. Bioresour. Technol. 100, 3650–3656. doi:10.1016/j.biortech.2009.02.029
Liang, R., Li, Y., Huang, L., Wang, X., Hu, X., Liu, C., et al. (2020). Pb2+ adsorption by ethylenediamine-modified pectins and their adsorption mechanisms. Carbohydr. Polym. 234, 115911. doi:10.1016/j.carbpol.2020.115911
Liu, W., Dong, Z., Sun, D., Chen, Y., Wang, S., Zhu, J., et al. (2019). Bioconversion of kitchen wastes into bioflocculant and its pilot-scale application in treating iron mineral processing wastewater. Bioresour. Technol. 288, 121505. doi:10.1016/j.biortech.2019.121505
Maliehe, T. S., Basson, A. K., and Dlamini, N. G. (2019). Removal of pollutants in mine wastewater by a non-cytotoxic polymeric bioflocculant from Alcaligenes faecalis HCB2. Int. J. Environ. Res. Public Health. 16 (20), 4001. doi:10.3390/ijerph16204001
Mohammed, J. N., and Dagang, W. R. Z. W. (2019). Implications for industrial application of bioflocculant demand alternatives to conventional media: Waste as a substitute. Water Sci. Technol. 80 (10), 1807–1822. doi:10.2166/wst.2020.025
Nguyen, C. V., Nguyen, A. V., Doi, A., Dinh, E., Nguyen, T. V., Ejtermaei, M., et al. (2021). Advanced solid-liquid separation for dewatering fine coal tailings by combining chemical reagents and solid bowl centrifugation. Sep. Purif. Technol. 259, 118172. doi:10.1016/j.seppur.2020.118172
Okaiyeto, K., Nwodo, U. U., Okoli, S. A., Mabubya, L. V., and Okoh, A. I. (2016). Implications for public health demands alternatives to inorganic and synthetic flocculants: Bioflocculants as important candidates. Microbiologyopen 5 (2), 177–211. doi:10.1002/mbo3.334
Poorgholy, N., Massoumi, B., and Jaymand, M. (2017). A novel starch-based stimuli-responsive nanosystem for theranostic applications. J. Biol. Macromol. 97, 654–661. doi:10.1016/j.ijbiomac.2017.01.063
Potgieter-Vermaak, S. S., Potgieter, J. H., Monama, P., and Grieken, R. V. (2006). Comparison of limestone, dolomite and fly ash as pre-treatment agents for acid mine drainage. Min. Eng. 19, 454–462. doi:10.1016/j.mineng.2005.07.009
Pu, L., Zeng, Y. J., Xu, P., Li, F. Z., Zong, M. H., Yang, J. G., et al. (2020). Using a novel polysaccharide BM2 produced by Bacillus megaterium strain PL8 as an efficient bioflocculant for wastewater treatment. Int. J. Biol. Macromol. 162, 374–384. doi:10.1016/j.ijbiomac.2020.06.167
Pu, S., Ma, H., Deng, D., Xue, S., Zhu, R., Zhou, Y., et al. (2018). Isolation, identification, and characterization of an Aspergillus niger bioflocculant-producing strain using potato starch wastewater as nutrilite and its application. PloS one 13 (1), e0190236. doi:10.1371/journal.pone.0190236
Rajivgandhi, G., Vimala, R., Maruthupandy, M., Alharbi, N. S., Kadaikunnan, S., Khaled, J. M., et al. (2021). Enlightening the characteristics of bioflocculant of endophytic actinomycetes from marine algae and its biosorption of heavy metal removal. Environ. Res. 200, 111708. doi:10.1016/j.envres.2021.111708
Saba, R. Y., Ahmed, M., and Sabri, A. N. (2019). Potential role of bacterial extracellular polymeric substances as biosorbent material for arsenic bioremediation. Bioremediat. J. 23 (2), 72–81. doi:10.1080/10889868.2019.1602107
Salaberria, A. M., Labidi, J., and Fernandes, S. C. M. (2014). Chitin nanocrystals and nanofibers as nano-sized fillers into thermoplastic starch-based biocomposites processed by melt-mixing. Chem. Eng. J. 256, 356–364. doi:10.1016/j.cej.2014.07.009
Salehizadeh, H., Vossoughi, M., and Alemzadeh, I. (2000). Some investigations on bioflocculant producing bacteria. Biochem. Eng. J. 5 (1), 39–44. doi:10.1016/S1369-703X(99)00066-2
Shahadat, M., Teng, T. T., Rafatullah, M., Shaikh, Z. A., Sreekrishnan, T. R., and Ali, S. W. (2017). Bacterial bioflocculants: A review of recent advances and perspectives. Chem. Eng. J. 328, 1139–1152. doi:10.1016/j.cej.2017.07.105
Sheng, Y., Zhang, Q., Sheng, Y., Li, C., and Wang, H. (2016). Screening and flocculating properties of bioflocculant-producing microorganisms. J. Univ. Sci. Technol. B 13 (4), 289–292. doi:10.1016/S1005-8850(06)60061-3
Sun, J., Zhang, X., Miao, X., and Zhou, J. (2012). Preparation and characteristics of bioflocculants from excess biological sludge. Bioresour. Technol. 126, 362–366. doi:10.1016/j.biortech.2012.08.042
Sun, P., Hui, C., Bai, N., Yang, S., Wan, L., Zhang, Q., et al. (2015). Revealing the characteristics of a novel bioflocculant and its flocculation performance in Microcystis aeruginosa removal. Sci. Rep. 5, 17465–17512. doi:10.1038/srep17465
Tawila, S. Z. M. M., Ismail, S., Amr, S. S. A., and Elkhair, E. K. A. (2019). A novel efficient bioflocculant QZ-7 for the removal of heavy metals from industrial wastewater. RSC Adv. 9 (48), 27825–27834. doi:10.1039/C9RA04683F
Tawila, Z. M. A., Ismail, S., Dadrasnia, A., and Usman, M. M. (2018). Production and characterization of a bioflocculant produced by Bacillus salmalaya 139SI-7 and its applications in wastewater treatment. Molecules 23 (10), 2689. doi:10.3390/molecules23102689
Vital, B., Bartacek, J., Ortega-Bravo, J. C., and Jeison, D. (2018). Treatment of acid mine drainage by forward osmosis: Heavy metal rejection and reverse flux of draw solution constituents. Chem. Eng. J. 332, 85–91. doi:10.1016/j.cej.2017.09.034
Wang, Z., Shen, L., Zhuang, X., Shi, J., Wang, Y., He, N., et al. (2015). Flocculation characterization of a bioflocculant from Bacillus licheniformis. Ind. Eng. Chem. Res. 54 (11), 2894–2901. doi:10.1021/ie5050204
Wu, H., Li, Q., Lu, R., Wang, Y., Zhuang, X., and He, N. (2010). Fed-batch production of a bioflocculant from Corynebacterium glutamicum. J. Ind. Microbiol. Biotechnol. 37, 1203–1209. doi:10.1007/s10295-010-0767-9
Xia, M., Zhou, H., Amanze, C., Hu, L., Shen, L., Yu, R., et al. (2022). A novel polysaccharides-based bioflocculant produced by Bacillus subtilis ZHX3 and its application in the treatment of multiple pollutants. Chemosphere 289, 133185. doi:10.1016/j.chemosphere.2021.133185
Xia, X., Lan, S., Li, X., Xie, Y., Liang, Y., Yan, P., et al. (2018a). Characterization and coagulation-flocculation performance of a composite flocculant in high-turbidity drinking water treatment. Chemosphere 206, 701–708. doi:10.1016/j.chemosphere.2018.04.159
Xia, X., Liang, Y., Lan, S., Li, X., Xie, Y., and Yuan, W. (2018b). Production and flocculating properties of a compound biopolymer flocculant from corn ethanol wastewater. Bioresour. Technol. 247, 924–929. doi:10.1016/j.biortech.2017.10.003
Yan, L., Kong, L., Qu, Z., Liang, L., and Shen, G. (2015). Magnetic biochar decorated with ZnS nanocrytals for Pb (II) removal. ACS Sustain. Chem. Eng. 3 (1), 125–132. doi:10.1021/sc500619r
Yang, Y. F., Yu, X., Zhu, Y., Zeng, Y., Fang, C., Liu, Y., et al. (2022). Preparation and application of a colorimetric film based on sodium alginate/sodium carboxymethyl cellulose incorporated with rose anthocyanins. Food Chem. 393, 133342. doi:10.1016/j.foodchem.2022.133342
Yang, Z., Wang, W., and Liu, S. (2017). Flocculation of coal waste slurry using bioflocculant produced by Azotobacter chroococcum. Energy Fuel. 31 (2), 1460–1467. doi:10.1021/acs.energyfuels.6b03052
Yu, L., Tang, Q., Zhang, Y., Chen, R., Liu, X., Qiao, W., et al. (2016). A novel Fe (III) dependent bioflocculant from Klebsiella oxytoca GS-4-08: Culture conditions optimization and flocculation mechanism. Sci. Rep. 6, 34980. doi:10.1038/srep34980
Zhang, X., Sun, J., Liu, X., and Zhou, J. (2013). Production and flocculating performance of sludge bioflocculant from biological sludge. Bioresour. Technol. 146, 51–56. doi:10.1016/j.biortech.2013.07.036
Zheng, Y., Ye, Z. L., Fang, X. L., Li, Y. H., and Cai, W. M. (2008). Production and characteristics of a bioflocculant produced by Bacillus sp. F19. Bioresour. Technol. 99, 7686–7691. doi:10.1016/j.biortech.2008.01.068
Keywords: bioflocculant, Corynebacterium glutamicum, structural characterization, flocculating activity, acid mine wastewater
Citation: Liu Y, Zeng Y, Yang J, Chen P, Sun Y, Wang M and Ma Y (2023) A bioflocculant from Corynebacterium glutamicum and its application in acid mine wastewater treatment. Front. Bioeng. Biotechnol. 11:1136473. doi: 10.3389/fbioe.2023.1136473
Received: 03 January 2023; Accepted: 15 February 2023;
Published: 28 February 2023.
Edited by:
Selin Şahin Sevgili, Istanbul University, TürkiyeReviewed by:
Vivek Rangarajan, Birla Institute of Technology and Science, IndiaChunqiao Xiao, Wuhan Institute of Technology, China
Mehmet Torun, Akdeniz University, Türkiye
Copyright © 2023 Liu, Zeng, Yang, Chen, Sun, Wang and Ma. This is an open-access article distributed under the terms of the Creative Commons Attribution License (CC BY). The use, distribution or reproduction in other forums is permitted, provided the original author(s) and the copyright owner(s) are credited and that the original publication in this journal is cited, in accordance with accepted academic practice. No use, distribution or reproduction is permitted which does not comply with these terms.
*Correspondence: Yan Zeng, zeng_y@tib.cas.cn; Yanhe Ma, ma_yh@tib.cas.cn