A novel human multiple myeloma cell line with a 1q21 gain genetic abnormality and CKS1B overexpression
Highlight box
Key findings
• Novel cell line with a 1q21 gain only was established.
What is known and what is new?
• Permanent MM cell lines act as effective tools.
• A novel MM cell line, CZ2, which is characterized by only 1q21 gain and CKS1B overexpression was established.
What is the implication, and what should change now?
• CZ2 is an important model for studies of myeloma cell growth.
Introduction
Multiple myeloma (MM) is a hematopoietic disease that is characterized by the proliferation of plasma cells in the bone marrow (1). In recent decades, protease inhibitors, immunomodulators, autologous stem cell transplantation, and several elective treatment strategies have been developed to improve the prognosis of MM (2), and the median survival time has been extended to 5–7 years (3). However, MM is still considered an incurable disease, as patients inevitably relapse after a variable time of following therapy (4).
The incurability of MM is closely related to its biological characteristics. The International Myeloma Work Group (IMWG) suggested that molecular biological anomaly markers such as 1q21 (gain), translocation t(4;14), and del(17p) can be applied for the risk-stratification of MM patients (5-7). Among these cytogenetic abnormalities, 1q21 gain (≤3 copy numbers) or amplification (>3 copy numbers), are relatively common types in MM patients (8). A 1q21 gain or amplification occurs in 30–40% of MM patients at the initial diagnosis and in 70% of patients at recurrence (9-11).
Numerous clinical studies have demonstrated that patients with 1q21 gain or amplification exhibit poor prognosis (12). Moreover, various genes located in 1q21 are involved in MM, especially CDC28 protein kinase regulatory subunit 1B (CKS1B), which is overexpressed in malignant tumors including MM, and is thought to be a factor of poor prognosis in refractory/recurrent MM patients (13,14). Collecting a bulk of neoplastic cells from MM patients for research is an inconvenient and time-consuming process. Therefore, determining the underlying mechanism of MMs with 1q21 gain or amplification is critical for the development of precise and efficient treatments to cure MM patients. For this, permanent malignant hematopoietic cell lines, which act as effective tools, are needed. In general, immortalized cell lines are capable of renewing themselves in artificial culture indefinitely. In the present study, we established a novel MM cell line, CZ2, which is characterized by only 1q21 gain, in hope that it can be utilized in MM research and the development of more effective therapies. We present the following article in accordance with the MDAR reporting checklist (available at https://atm.amegroups.com/article/view/10.21037/atm-22-5741/rc).
Methods
Patient
The study was conducted in accordance with the Declaration of Helsinki (as revised in 2013). The study was approved by the Ethics Committee of Changzheng Hospital (No. AF-HEC-055) and informed consent was obtained from the patient. Our patient was a 70-year-old male who was diagnosed with stage III MM according to the International Staging System (ISS) and stage IIIA MM based on the Durie-Salmon (DS) staging system. Bone marrow aspiration showed that plasma cells accounted for 97.5% of the bone marrow cells. The patient’s myeloma cells secreted a monoclonal protein of immunoglobulin D (IgD), the kappa chain. Also, his serum immunoglobulin G (IgG) was 3.39 g/L, immunoglobulin A (IgA) was 0.26 g/L, and immunoglobulin M (IgM) was 0.18 g/L. For this experiment, we used pleural effusion cells at the cell collection stage.
Cell culture and establishment of cell line
Pleural effusion specimens were obtained from the patient and the mononuclear cells were separated by Ficoll density sedimentation. CD138+ cells were isolated using CD138 magnetic beads and cultured in RPMI-1640 medium (Gibco, USA) supplemented with 10% fetal bovine serum (FBS; Gibco, USA), penicillin (100 U/mL), and streptomycin (100 µg/mL). The cells were cultured in an incubator under an atmosphere with 5% carbon dioxide (CO2) at 37 °C, and the culture medium was exchanged every 2–3 days to maintain optimal cell growth.
Morphology and cytogenetic analysis
Cytospin preparations were routinely prepared and harshly treated (87 °C for 10 minutes, then cooled to 70 °C), and then stained using Giemsa to regularly assess the cell type morphology and the presence of mitoses. Cell morphology was examined using light microscopy.
Fluorescence in situ hybridization (FISH) analysis
FISH was performed on interphase CZ2 using a Fluorescent in situ Hybridization Kit (Cytocell, UK), according to the manufacturer’s instructions. The commercial FISH probes targeting 1q21, 13q14 (RB1 gene), 17p13 (TP53 gene), and translocation t(4;14) were used for this assay. The probe used in the FISH test was obtained from the p53/D13S319/RB1/1q21/IgH/(IgH/CCND1)/(IgH/MAF)/(IgH/MAFB)/(IgH/FGFR3) DNA Probe kit (F.01367-01, AnBiPing, China). At least 200 interphase cells with intact nuclei were evaluated for each probe. Positive nuclear staining in more than 20% of the cells was considered positive.
Chromosomal microarray analysis (CMA)
The experiment was performed as previously reported (15). Hybridization-based genomic profiling arrays were blindly performed on the CytoSan HD array platform, which contains 2.6 million and 1.8 million combined copy number variation (CNV) and single nucleotide polymorphism (SNP) markers, respectively, with a median inter-marker distance of 500–600 bases (Affymetrix, Inc., Santa Clara, CA, USA). Hybridizations were performed according to the manufacturer’s protocols. CEL files obtained from the CytoScan HD array platform were analyzed using the Chromosome Analysis Suite software package (Affymetrix) and the Nexus copy number software (Biodiscovery Inc., Hawthorne, CA, USA). The GRCh37 (hg19) genome version was applied for annotations. Measures achieving the manufacturer’s quality cut-off -were included in the analysis.
Immunophenotypic analysis by flow cytometry
The following antibodies were used for immunophenotypic characterization of the CZ2 cells: CD1a, CD19, CD20, CD21, CD25, CD3, CD28, CD33, CD38, CD45, CD56, CD117, CD138, CD4, CD13, CD27, CD5, CD8, CD9, CD10, CD24, CD15, CD34, CD44, CD89, CD95, CD98, CD96, CD105, CD184, HLA-DR; and cytoplasmic κ and γ light immunoglobulin chains (Invitrogen, USA).
Immunoglobulin secretion
To measure the secretion of free immunoglobulin light chains in the serum and culture medium of CZ2, the nephelometric technique was applied and performed on a Siemens BNII Nephelometer (Siemens Healthcare Ltd., Newark, DE, USA).
Virus detection
The presence of Epstein-Barr virus (EBV) was examined using an EBV polymerase chain reaction (PCR) Fluorescence Detection Kit (Huirui Biotechnology, Shanghai, China). PCR was carried out using a LightCycler480 II (Roche, USA) for 40 cycles.
Western blotting analysis
The whole cell protein from CZ2 and other MM cells was collected and resolved in 10% sodium dodecyl sulfate-polyacrylamide gel electrophoresis (SDS-PAGE) gels, and then electrotransferred to polyvinylidene difluoride membranes (Millipore, USA). Rabbit polyclonal anti-CKS1B antibody (1:500, Invitrogen, CA, USA), rabbit polyclonal anti-poly(ADP-ribose) polymerase (anti-PARP) antibody (1:1,000, Beyotime Biotech. Inc., Shanghai, China), and rabbit polyclonal anti-caspase3 antibody (1:1,000, Beyotime Biotech. Inc., Shanghai, China) were used as primary antibodies, while horseradish peroxidase-conjugated goat anti-rabbit IgGs (1:3,000, Santa Cruz, CA, USA) were used as secondary antibodies. After treatment with the secondary antibodies, the protein bands were detected by enhanced chemiluminescence.
Cell viability analysis
The 3-(4,5-dimethyl thiazol-2-yl)-2,5-diphenyl tetrazolium bromide (MTT) assay was used to measure the cell viability of CZ2 and other MM cells. These cells were seeded in 96-well plates and transfected with CKS1B small interfering RNA (siRNA) or control siRNA (Shanghai GenePharma Co., Ltd., Shanghai, China). The target sequences of siRNAs are listed in Supplemental Table 1. After 48 or 72 h of treatment, 10 µL of the MTT viability reagent was added to each well. The plates were incubated in an incubator at 37 °C for 2 h. The medium was then discarded and 200 µL dimethyl sulfoxide (DMSO) was added to each well. The absorbance at 570 nm was then determined with a microplate reader (Tecan, Spark, Switzerland).
Table 1
Location | Cytogenetic abnormality | Copy number |
---|---|---|
Chromosome 1 | dup(1)(p36.33p34.3) | 3 |
dup(1)(p34.3p32.3) | 4 | |
dup(1)(p32.3p13.3) | 3 | |
dup(1)(p13.3p12) | 4 | |
dup(1)(p11.2q23.3) | 3 | |
dup(1)(q23.3q25.3) | 4 | |
dup(1)(q25.3q44) | 3 | |
Chromosome 2 | dup(2)(p25.3q23.2) | 3 |
dup(2)(q23.2q32.1) | 4 | |
hmz(2)(q32.1q37.3) | – | |
Chromosome 3 | dup(3)(p26.3p26.1) | 3 |
dup(3)(p26.1p25.1) | 4 | |
dup(3)(p25.1p21.31) | 3 | |
hmz(3)(p26.3q29) | – | |
Chromosome 4 | dup(4)(p16.3q32.1) | 3 |
del(4)(q32.1q32.2) | 1 | |
dup(4)(q32.2q35.2) | 3 | |
Chromosome 5 | dup(5)(p15.33q11.2) | 4 |
dup(5)(q11.2q31.3) | 3 | |
dup(5)(q32q34) | 3 | |
dup(5)(q34q35.3) | 3 | |
Chromosome 6 | dup(6)(p25.3p12.3) | 4 |
dup(6)(p12.3q13) | 3 | |
dup(6)(q13q15) | 4 | |
dup(6)(q23.3q27) | 3 | |
hmz(6)(6q15q23.3) | – | |
Chromosome 7 | dup(7)(p22.3p11.2) | 3 |
dup(7)(q11.21q36.3) | 4 | |
Chromosome 8 | dup(8)(p23.3p22) | 3 |
dup(8)(p11.23q24.3) | 3 | |
hmz(8)(p23.3p11.23) | – | |
Chromosome 9 | del(9)(p21.3p21.2) | 0 |
del(9)(p21.1) | 0 | |
dup(9)(p21.1p13.2) | 3 | |
dup(9)(p13.2p13.1) | 5 | |
dup(9)(q22.31q34.3) | 3 | |
hmz(9)(p24.3p13.1) | – | |
hmz(9)(q21.11q34.3) | – | |
Chromosome 10 | dup(10)(p15.3p12.1) | 3 |
dup(10)(p12.1p11.23) | 4 | |
dup(10)(p11.23q21.1) | 3 | |
dup(10)(q21.1) | 4 | |
dup(10)(q21.1q23.1) | 3 | |
hmz(10)(q23.1q26.3) | – | |
Chromosome 11 | dup(11)(p15.5p14.2) | 3 |
dup(11)(p14.2p14.1) | 4 | |
dup(11)(p14.1q14.1) | 3 | |
dup(11)(q14.1q25) | 4 | |
Chromosome 12 | dup(12)(p12.1q24.33) | 3 |
hmz(12)(p13.33p12.1) | – | |
Chromosome 13 | dup(13)(q31.3) | 13 |
hmz(13)(q11q34) | – | |
Chromosome 14 | dup(14)(q24.2q32.33) | 3 |
hmz(14)(q11.2q32.33) | – | |
Chromosome 15 | dup(15)(q25.1q26.3) | 3 |
Chromosome 16 | dup(16)(p13.3q23.1) | 3 |
dup(16)(q23.1) | 4 | |
dup(16)(q23.1q24.1) | 5 | |
dup(16)(q24.1q24.3) | 4 | |
Chromosome 17 | dup(17)(q11.1q25.3) | 3 |
hmz(17)(p13.3p11.2) | – | |
Chromosome 18 | dup(18)(p11.32p11.22) | 4 |
dup(18)(p11.22q11.2) | 3 | |
dup(18)(q11.2q12.1) | 5 | |
dup(18)(q12.1q12.3) | 4 | |
Chromosome 19 | dup(19)(p13.3q13.43) | 3 |
Chromosome 20 | dup(20)(q11.1q13.33) | 3 |
hmz(20)(p13p11.1) | – | |
Chromosome 21 | dup(21)(q11.2q21.2) | 3 |
dup(21)(q21.2q22.3) | 4 | |
Chromosome 22 | dup(22)(q11.1q11.21) | 4 |
dup(22)(q11.21) | 11 | |
dup(22)(q11.21q11.23) | 13 | |
hmz(22)(q11.23q13.33) | – | |
Chromosome X | dup(X)(p22.33) | 3 |
hmz(X)(p22.33q28) | – |
CMA, chromosomal microarray analysis.
Statistical analysis
All experiments were independently replicated at least three times with replicate samples. Statistical analysis was performed using the Student’s t-test or paired t-test.
Results
Establishment and biological characterization of the CZ2 MM cell line
The separated cells from the patient’s pleural effusion were cultured in RPMI-1640 supplemented with 10% FBS and grown as single cells or in small clusters in a suspension. The cell doubling time was about 48 h; hence, they could be passaged every 2 or 3 days. Following 20 months (68 passages), the CZ2 cell line displayed stable proliferation. The cells were then frozen in liquid nitrogen and proliferated after revival. Using FACScan (Beckman Coulter, CA, USA), we found that in the exponential growth phase, 44.72% of the cells were in the G1 phase, 5.93% were in the G2 phase, and 49.36% were in the S phase (Figure 1). Wright-Giemsa staining showed that the cells exhibited a typical plasma cell morphology, which had large nuclei, 2–3 nuclei/cell, and a basophilic cytoplasm (Figure 2).
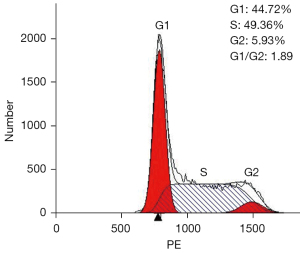
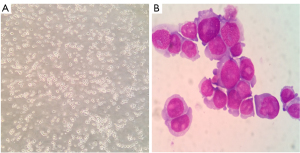
Immunophenotypic and immunoglobulin analysis
The CZ2 cells did not express CD38, which is a typical plasma cell phenotype. In addition, the expressions of CD19, CD45, and CD117 were negative, whereas those of CD43, CD28, CD40, CD58, and β2 were positive. Cells were partly positive for CD10 and c-MYC and marginally positive for CD7, CD15, and CyclinD1. Moreover, the CZ2 cells were also found to be non-secretory cells after several generations. Furthermore, the PCR results of the cells showed them to be EBV-negative.
Cytogenetic characterization
Classic cytogenetic analysis revealed that 65–67 chromosomes were found with the following aberrations in the 41st generation of the cells: 65~67<3n>,XX,+1,+del(1)(q?),+del(1)(p?),+2,+5,+5,+6,+7,+7,+del( 7)(p?),+del(8)(p?),9p+,+del(9)(q?),+10,+10,+del(10)(q?),+11,+11,+12,+12,-13,-14,+15,+16,+16,+del(17)(p?),+del(17)(p?),del(18)(q?),+19,+21(cp10) (Figure 3).
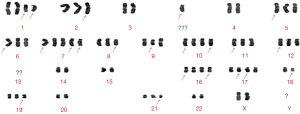
Given that FISH results have been included in the Revised International Staging System (RISS), FISH analysis is necessary during the diagnosis and treatment of MM. Using the common MM probes [i.e., the 1q21 probe, the TP53/CEP 17 FISH probe, the D13S319 (13q14.3) probe, and the immunoglobulin heavy chain (IgH) dual color probe], we found that the only cytogenetic abnormality was a 1q21 region (1q21+) gain; no other common cytogenetic abnormalities in MM, such as deletion of 17p (17p-), deletion of 13q (13q-), and translocation of IgH (Figure 4), were observed.
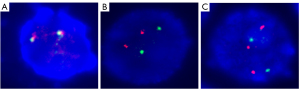
In addition, CMA pinpointed various pathogenic CNVs in the CZ2 cell line (Table 1), including the CNVs in 1q21. Furthermore, the CMA also confirmed that there were no other common cytogenetic abnormalities of MM, such as 17p-, 13q-, and translocation of IgH was present in the CZ2 cell line.
Molecular characterization
Considering that the MM cell line CZ2 exhibited 1q21+ as the only cytogenetic abnormality, we focused on the 1q21 region of the cells. There are numerous MM-associated genes located in the 1q21 chromosomal region, among which CKS1B is the most widely studied, and is a promising immunotherapeutic target of MM. Through western blotting analysis, we found that the CKS1B protein expression in CZ2 cells was significantly higher than that in peripheral blood mononuclear cells (PBMCs) of normal people (Figure 5). Meanwhile, compared with the other MM cell line RPMI 8226, CZ2 cells expressed higher levels of the CKS1B protein (Figure 5).
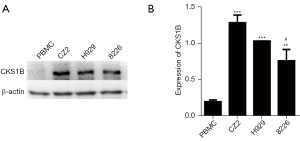
To explore the role of CKS1B in MM cells, we knocked down the expression of CKS1B by siRNA technology (Table S1) in the CZ2, H929, and RPMI 8226 cell lines (Figure 6A). The MTT assay results revealed that cell viability was significantly impaired in RPMI 8226, H929, and CZ2 after 48 h of CKS1B siRNA interference, and in H929 and CZ2 after 72 h of CKS1B siRNA interference, indicating that CKS1B is vital for MM cell survival (Figure 6B).
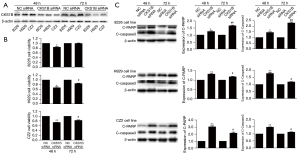
To investigate the role of CKS1B in cell apoptosis, we examined the expressions of cleaved-PARP and cleaved-caspase3. Our results showed that the expression of cleaved-caspase3 and cleaved-PARP significantly increased in the CKS1B-knockdown CZ2, H929, and RPMI 8226 cell lines (n=3) (P<0.05), suggesting that the overexpression of CKS1B reduced cell apoptosis (Figure 6C).
Discussion
In this study, we established a unique myeloma cell line, CZ2, which possesses the genetic abnormality of 1q21 gains only, from the pleural effusion of a patient with MM. The CZ2 cells were found to be non-immunoglobulin-secretary cells. Meanwhile, through western blotting, we also observed that CKS1B, an adverse prognostic gene that is located in the 1q21 region, was highly expressed in CZ2 (Figure 5). Knockdown of CKS1B reduced cell viability and increased the levels of cleaved-PARP and cleaved-caspase3 (Figure 6). It has been reported that several MM cell lines are eventually transformed into original lymphoid cell lines due to EBV infection (16,17). Using PCR, we confirmed that CZ2 are EBV-negative cells. Drexler et al. proved that the typical MM cells highly express CD138, CD38, CD28, and CD58, and negatively express CD10 and CD19 (16). However, we observed that CZ2 did not express CD38.
Cell lines represent a convenient and readily available model to explore disease mechanisms, especially tumors. Many advanced understandings of MM and other malignant hematopathies have been achieved using immortal hematopathic cell lines. At present, more than 100 MM cell lines have been established (18). Nonetheless, various characteristics of the cell lines have not been probed clearly due to technological limitations at the time, especially cytogenetic and molecular characteristics. Moreover, cytogenetic analysis results have shown that numerous MM cell lines exhibit complicated cytogenetic abnormalities. In contrast, our CZ2 cell line not only has detailed cytogenetic results detected by karyotyping and FISH, but also only exhibits 1q21 gains, without other common MM genetic abnormalities. Therefore, CZ2 is an ideal model to study the underlying mechanism of 1q21 gains in MM.
Immunotherapy is thought to be a promising treatment for tumors including MM (19,20). Exploring a suitable tumor-specific antigen, which is expressed in tumors but not in other normal tissues, or a tumor-associated antigen, which is overexpressed in tumors but poorly expressed in normal cells, is crucial in active immunotherapy. Notably, CKS1B, MCL1, IL6R, BCL9, ILF2, as well as several MM-associated antigens are located in the 1q21 chromosomal region. In this study, we found that CKS1B was overexpressed in the CZ2 and other MM cell lines (Figure 5); in addition, CKS1B-knockdown decreased cell viability (Figure 6).
The role of CKS1B in MM has been explored for a long time. Bock et al. reported that the median progression-free survival (PFS) of the CKS1B and the control groups were 15.0 and 33.0 months (P=0.002), respectively (21). Moreover, CKS1B was thought to be an adverse prognostic factor in refractory or recurrent MM (13). Zhan et al. and Huang et al. found that the overexpression of CKS1B in MM could increase the degradation of cyclin-dependent kinase inhibitors, such as P27 and P21, concluding that CKS1B induced the growth and drug resistance of MM (22,23).
Conclusions
In summary, we established CZ2, which is a novel human MM cell line with only 1q21 gains, providing a characteristic model for pathological and therapeutic research of MM. The unique chromosomal abnormalities of CZ2 allow for this cell line to be utilized to better understand the genetic and molecular mechanisms of 1q21 gains in MM.
Acknowledgments
Funding: This work was supported by the National Natural Science Foundation of China (No. 81870159), the Shanghai Pujiang Talent Program (No. 18PJD059), and the Pyramid Talent Program of Changzheng Hospital.
Footnote
Reporting Checklist: The authors have completed the MDAR reporting checklist. Available at https://atm.amegroups.com/article/view/10.21037/atm-22-5741/rc
Data Sharing Statement: Available at https://atm.amegroups.com/article/view/10.21037/atm-22-5741/dss
Conflicts of Interest: All authors have completed the ICMJE uniform disclosure form (available at https://atm.amegroups.com/article/view/10.21037/atm-22-5741/coif). The authors have no conflicts of interest to declare.
Ethical Statement: The authors are accountable for all aspects of the work in ensuring that questions related to the accuracy or integrity of any part of the work are appropriately investigated and resolved. The study was conducted in accordance with the Declaration of Helsinki (as revised in 2013). The study was approved by the Ethics Committee of Changzheng Hospital (No. AF-HEC-055) and informed consent was taken from the patient.
Open Access Statement: This is an Open Access article distributed in accordance with the Creative Commons Attribution-NonCommercial-NoDerivs 4.0 International License (CC BY-NC-ND 4.0), which permits the non-commercial replication and distribution of the article with the strict proviso that no changes or edits are made and the original work is properly cited (including links to both the formal publication through the relevant DOI and the license). See: https://creativecommons.org/licenses/by-nc-nd/4.0/.
References
- Bernstein ZS, Kim EB, Raje N. Bone Disease in Multiple Myeloma: Biologic and Clinical Implications. Cells 2022;11:2308. [Crossref] [PubMed]
- van de Donk NWCJ, Pawlyn C, Yong KL. Multiple myeloma. Lancet 2021;397:410-27. [Crossref] [PubMed]
- Dima D, Jiang D, Singh DJ, et al. Multiple Myeloma Therapy: Emerging Trends and Challenges. Cancers (Basel) 2022;14:4082. [Crossref] [PubMed]
- Avet-Loiseau H, Bahlis NJ, Chng WJ, et al. Ixazomib significantly prolongs progression-free survival in high-risk relapsed/refractory myeloma patients. Blood 2017;130:2610-8. [Crossref] [PubMed]
- Palumbo A, Avet-Loiseau H, Oliva S, et al. Revised International Staging System for Multiple Myeloma: A Report From International Myeloma Working Group. J Clin Oncol 2015;33:2863-9. [Crossref] [PubMed]
- Hanamura I. Multiple myeloma with high-risk cytogenetics and its treatment approach. Int J Hematol 2022;115:762-77. [Crossref] [PubMed]
- Paner A, Patel P, Dhakal B. The evolving role of translocation t(11;14) in the biology, prognosis, and management of multiple myeloma. Blood Rev 2020;41:100643. [Crossref] [PubMed]
- Pawlyn C, Morgan GJ. Evolutionary biology of high-risk multiple myeloma. Nat Rev Cancer 2017;17:543-56. [Crossref] [PubMed]
- Mei J, Zhai Y, Li H, et al. Prognostic impact of hyperdiploidy in multiple myeloma patients with high-risk cytogenetics: a pilot study in China. J Cancer Res Clin Oncol 2018;144:2263-73. [Crossref] [PubMed]
- An G, Xu Y, Shi L, et al. Chromosome 1q21 gains confer inferior outcomes in multiple myeloma treated with bortezomib but copy number variation and percentage of plasma cells involved have no additional prognostic value. Haematologica 2014;99:353-9. [Crossref] [PubMed]
- Klein U, Jauch A, Hielscher T, et al. Chromosomal aberrations +1q21 and del(17p13) predict survival in patients with recurrent multiple myeloma treated with lenalidomide and dexamethasone. Cancer 2011;117:2136-44. [Crossref] [PubMed]
- Bisht K, Walker B, Kumar SK, et al. Chromosomal 1q21 abnormalities in multiple myeloma: a review of translational, clinical research, and therapeutic strategies. Expert Rev Hematol 2021;14:1099-114. [Crossref] [PubMed]
- Chen MH, Qi C, Reece D, et al. Cyclin kinase subunit 1B nuclear expression predicts an adverse outcome for patients with relapsed/refractory multiple myeloma treated with bortezomib. Hum Pathol 2012;43:858-64. [Crossref] [PubMed]
- Hanamura I. Gain/Amplification of Chromosome Arm 1q21 in Multiple Myeloma. Cancers (Basel) 2021;13:256. [Crossref] [PubMed]
- Li C, Wendlandt EB, Darbro B, et al. Genetic Analysis of Multiple Myeloma Identifies Cytogenetic Alterations Implicated in Disease Complexity and Progression. Cancers (Basel) 2021;13:517. [Crossref] [PubMed]
- Drexler HG, Matsuo Y. Malignant hematopoietic cell lines: in vitro models for the study of multiple myeloma and plasma cell leukemia. Leuk Res 2000;24:681-703. [Crossref] [PubMed]
- Drexler HG, Matsuo Y, MacLeod RA. Persistent use of false myeloma cell lines. Hum Cell 2003;16:101-5. [Crossref] [PubMed]
- Kumar SK, Rajkumar V, Kyle RA, et al. Multiple myeloma. Nat Rev Dis Primers 2017;3:17046. [Crossref] [PubMed]
- Rendo MJ, Joseph JJ, Phan LM, et al. CAR T-Cell Therapy for Patients with Multiple Myeloma: Current Evidence and Challenges. Blood Lymphat Cancer 2022;12:119-36. [Crossref] [PubMed]
- Goldsmith SR, Streeter S, Covut F. Bispecific Antibodies for the Treatment of Multiple Myeloma. Curr Hematol Malig Rep 2022;17:286-97. [Crossref] [PubMed]
- Bock F, Lu G, Srour SA, et al. Outcome of Patients with Multiple Myeloma and CKS1B Gene Amplification after Autologous Hematopoietic Stem Cell Transplantation. Biol Blood Marrow Transplant 2016;22:2159-64. [Crossref] [PubMed]
- Zhan F, Colla S, Wu X, et al. CKS1B, overexpressed in aggressive disease, regulates multiple myeloma growth and survival through SKP2- and p27Kip1-dependent and -independent mechanisms. Blood 2007;109:4995-5001. [Crossref] [PubMed]
- Huang J, Zhou Y, Thomas GS, et al. NEDD8 Inhibition Overcomes CKS1B-Induced Drug Resistance by Upregulation of p21 in Multiple Myeloma. Clin Cancer Res 2015;21:5532-42. [Crossref] [PubMed]
(English Language Editor: A. Kassem)