Abstract
Fabrication of large-area colloidal crystals with superb uniformity in multiple layers (
layers) from
microspheres
was achieved by electrophoresis in vertical arrangement. With a large difference in electrode areas and selection of ethanol as the solvent, we investigated self-assembly with
suspensions at various pH and electric fields of
. Colloid formations with negligible vacancies and small-angle grain boundaries were obtained at a suspension of
and electric field of
. Our fabrication scheme enables construction of colloidal crystals with significantly reduced structural disorders as compared to conventional routes.
Export citation and abstract BibTeX RIS
Fabrication of colloidal crystals by self-assembly has received significant attention recently because it provides an inexpensive approach to construct three-dimensional periodic structures for photonic crystals and biosensors.1–3 To date, methods including gravitational sedimentation, solvent evaporation, spatial confinement with topographic contrasts, and others have been investigated with various successes.4–15 The formation of colloidal crystals typically involves synthesis of microspherical or polystyrene latex followed by suspension and deposition onto a substrate to obtain structures with desirable packing orders and sizes.
In colloidal structure preparation, gravity plays an important role in the resulting crystal qualities. To minimize crystallographic defects, the fabrication process often takes weeks to finish to allow adequate time for colloid settlements. Unfortunately, excess formation time is impractical from a device fabrication standpoint. Therefore, for higher deposition rates many researchers have pursued the electrophoresis route.16–22 It is known that the colloids carry distinct surface charges in solvents and under an externally applied electric field, these charged colloids would drift and deposit on conductive substrates opposite to their polarity. This packing behavior can be disrupted once the electric field is reversed. It is rationalized that the electrophoretic force confers an alternative drive to the gravity in directing colloid movements, enabling rapid and designed crystal formations.
Earlier reports of electrophoretic deposition were conducted in aqueous solutions with electrodes aligned horizontally at a close distance (in the millimeter range) to avoid water electrolysis.21, 22 However, this setup adds considerable difficulties in sample retrieval, not to mention the interference of gravity in surface uniformities. In contrast, the electrodes arranged vertically are expected to incur much fewer challenges. Electrophoresis of polystyrene microspheres in a vertical electrode arrangement is straightforward for their reduced mass density, which helps in counteracting the influence of gravity. The microspheres reveal a much smaller processing window. For applications in the photonic crystals,
is preferred for its relatively high refractive index. Hence, for practical device fabrications rigorous controls over the deposition steps in large-area substrates become critical. In this work we demonstrate a large-area fabrication technique to prepare multilayer
colloidal crystals in a relatively short time
by the electrophoresis process in vertically arranged electrodes with uneven sizes. Ethanol was selected as the solvent, because unlike water, its low capillary pressure is expected to minimize the structure alteration during solvent evaporation after the sample is removed. Furthermore, structural interruption by gas evolution from the water electrolysis is likely to be prevented once the ethanol is used to replace aqueous solvents.
Experimental
Monosized microspheres were fabricated by a sol-gel method where
of
tetraethoxysilane was mixed with
of
ethanol followed by addition of
of
to initiate the hydrolysis and condensation reactions. Afterward, an opaque
suspension was formed and maintained at
for
to acquire
microspheres with desirable sizes. Subsequently, the suspension was washed with deionized water and centrifuged to retrieve the
concentrate followed by drying at
for
. The as-synthesized
microspheres exhibited an average diameter of
with a standard deviation of
. In electrophoretic deposition,
of
microspheres was mixed in
of
ethanol followed by ultrasonication for
. A minute amount of
or
aqueous solution was added to adjust the pH of the
suspension between 1.37 and 10. The suspensions underwent zeta-potential measurements (Malvern Zetasizer Nano Zs) to record the values for zeta potential and electrophoretic mobility. The electrophoretic deposition was carried out with two electrodes aligned vertically facing each other. n-Type Si
was used as the electrodes. The areas for the working electrode (the substrate to be deposited) and counter electrode were 1 and
, respectively. The large difference in the electrode areas allows us to minimize the edge effect and promote a better surface uniformity in the deposited colloidal crystals. The distance between the electrodes was kept at
, while the voltage was varied between 20 and
, resulting in an electric field strength of
. Figure 1 exhibits the schematic diagram of the experimental setup. The electrophoretic deposition lasted for
. When finished, the sample was removed and kept for
at
to evaporate the ethanol slowly. Scanning electron microscopy (SEM, JEOL-JSM-6700) was employed to observe the morphologies of the colloidal crystals. The current incurred during the electrophoretic deposition was recorded by a potentiostat (EG&G 263A). A pycometer (Micromeritics AccuPyc 1340) was used to measure the density of the as-synthesized
microspheres.
Figure 1. A schematic diagram of the experimental setup.
Results and Discussion
Figure 2 provides the results of zeta potential and electrophoretic mobility for the suspensions at various pH values. It is understood that the zeta potential reflects the repulsive electrostatic forces among individual colloids, while the electrophoretic mobility represents their traveling abilities under the externally applied electric field. As clearly shown, the isoelectric point (point of zero charge) for the
microspheres was identified at pH 4.0, at which pronounced agglomerations and sedimentations occurred. Once the suspension was formulated to basic conditions
, we noticed much improved stabilities for the
microspheres, a fact attributed to their increasing zeta potentials. Correspondingly, their electrophoretic mobility rose as expected. We rationalized that for the precise control in the formation of colloidal crystals, a reasonable balance is necessary for the colloid stability and electrophoretic mobility.
Figure 2. The values of zeta potential and electrophoretic mobility for the microspheres at suspensions of various pH values.
We carried out the electrophoretic depositions at various pH values under electrical fields of 20, 40, and . Because the colloidal movement is proportional to their mobility and electric field, we expect to obtain nicely packed crystals under suitable combinations of both. After exploring many experimental conditions, we determined suspensions at pH 10 and electrical field of
to be the optimized parameters for the colloid formations. Previously, Holgado et al.23 pointed out that in suspensions the colloid settlement velocity
can be expressed by contributions from both gravity and electrophoresis
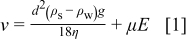
where is the diameter of the
microsphere,
and
are the mass densities for
microspheres and ethanol,
is the gravity acceleration, η is the viscosity of ethanol, μ is the electrophoretic mobility, and
is the externally applied electrical field. To succeed in the colloid deposition, the contributions from electrophoresis must surpass that of gravity. In our experiments, the gravity engenders a downward velocity of
, while the electrophoretic force produces a horizontal drift of
at an electric field of
. Hence, in our experimental conditions we conclude there was negligible contribution from gravity during electrophoresis in crystal formations.
The time evolutions of the electrophoretic current and deposited weight are demonstrated in Fig. 3. Apparently, the deposited weight exhibited an incubation stage for followed by a sharp increase, reaching a plateau after
. In contrast, the electrophoretic current started at the highest value and dropped considerably as time progressed. These responses were attributed to the screening effect of the depositing colloids that effectively reduces the magnitude of electric field. The details of this mechanism were explained by Besra and Liu.24 In our case, deliberate controls for the defectless colloid deposition was achieved for deposition times after
. The density of the as-synthesized
microspheres was measured at
. Therefore, we can deduce the degree of packing by taking into account the volume
and cross section
of each
microsphere to reach the mass required for a complete coverage at
. Table I lists the deposited weight and the estimated layers of the colloidal crystals.
Figure 3. The time dependence of current density and deposited weight during the electrophoretic deposition at pH 10 and .
Table I. The measured weight and estimated layers of colloidal crystals after electrophoretic deposition with suspensions of pH and .
Time (s) | 60 | 180 | 300 | 600 | 1200 | 1800 | 3600 | 5400 | 7200 |
---|---|---|---|---|---|---|---|---|---|
Measured weight ![]() | 0.74 | 0.74 | 0.75 | 1.62 | 3.45 | 12.5 | 49.2 | 76.8 | 78.2 |
Estimated layers |
![]() |
![]() |
![]() |
![]() |
![]() |
![]() |
![]() |
![]() |
![]() |
The SEM images of the assembled colloidal crystal after of deposition are shown in Fig. 4. Figures 4A and B exhibit the top views in high and low magnifications, respectively. From Table I it is estimated that 128 layers of colloids were deposited, which corresponds to a thickness of
. Excellent surface uniformity was observed without occurrence of islands and valleys. In the high-magnification picture, the colloidal crystal revealed a near-perfect hexagonal lattice (111), with the presence of a single vacancy. In contrast, at low magnification picture defects including vacancies and small-angle grain boundaries were evident. The areas for an average grain were approximately
, a value that is much larger than what were typically observed
in literature.4–8, 15–18, 25, 26 We believe the improved crystallinity likely resulted from our experimental design which makes available sufficient times and electric field force for the
microspheres to find the lowest energy sites. A point to emphasize is that the superb surface uniformity was observed for the colloids at the 128th layer. With a thickness of
and large-area grain size, the colloidal crystal fabricated in this route promises substantial potentials for device fabrications. The phenomenon of crystal formation in three-dimension was further confirmed by the image from a side view, as exhibited in Fig. 4C. This image does not represent the cross-sectional view but an oblique interface. Clearly, the colloidal crystal extended to multiple layers, as indicated by markers identifying both the (111) and (100) planes, and the total deposited
microspheres amounted to less than
of the
in suspensions even after
of deposition. So the limitation for the colloid deposition was simply due to the screening effect in electrophoresis, as evident from Fig. 3. In addition, we believe the base area of the colloidal crystals could be increased simply by scaling up the working and counter electrodes proportionally.
Figure 4. SEM images of the colloidal crystals after of electrophoretic deposition from the
suspensions with pH 10 and
; (A) top view at high magnification, (B) top view at low magnification, and (C) side view at low magnification.
Conclusion
In this study we demonstrated the fabrication of colloidal crystals by electrophoretic deposition in ethanol with uneven-sized electrodes in a vertical arrangement. With a process time of
, multiple layers of colloids (
layers) were assembled in grain sizes of
on substrates of
. The colloidal crystals revealed superb surface uniformity with significantly reduced defects as compared to those from conventional routes. Our fabrication scheme is simple and effective, enabling the construction of large-area photonic crystals in a reasonable timeframe.
Acknowledgments
The authors would like to thank the National Science Council of Taiwan for a financial grant (NSC-96-2221-E-009-110). An equipment loan from Professor Chihpin Huang of the Institute of Environmental Engineering is highly appreciated.
National Chiao Tung University assisted in meeting the publication costs of this article.