Abstract
Carbon-based electrode materials have been widely used for many years for electrochemical charge storage, energy generation, and catalysis. We have developed an electrode material with high specific capacitance by entrapping graphite nanoparticles in a sol-gel network. Films from the resulting colloidal suspensions were porous due to the removal of the entrapped organic solvents from the sol-gel matrix giving rise to high Brunauer–Emmett–Teller specific surface areas and a high capacitance density
. An exponential decrease of capacitance was observed with increasing scan rates in cyclic voltammetry studies on these films, suggesting the presence of pores ranging from micro-
to mesopores. Nitrogen adsorption–desorption analysis and scanning electron microscope images of these films also confirmed the presence of the micropores as well as mesopores. A steep drop in the double-layer capacitance with polar electrolytes was observed when the films were rendered hydrophilic upon exposure to mild oxygen plasma. We propose a model whereby the microporous hydrophobic sol-gel matrix perturbs the hydration of ions which moves ions closer to the graphite nanoparticles, consequently increasing the capacitance of the film.
Export citation and abstract BibTeX RIS
Carbon-based electrode materials are widely used in research and industry for a variety of applications. For example, high-porosity carbon matrices have been heavily explored in building ultracapacitors particularly due to their high-energy storage density and low cost.1 The energy storage density is prominent in these materials due to a large available surface area of the porous matrix. Some other applications include air and water purification, gas separation, catalysis, and chromatography. Most of these applications utilize exceptional properties of porous carbon materials such as high surface area, large pore volumes, chemical inertness, and good mechanical stability.2 In addition, carbon-based electrodes have desirable properties for electrochemistry, such as a large potential window between the water splitting reactions, low or constant background current, and fast electron-transfer kinetics.3
There are several routes to synthesis of porous carbon, such as pyrolysis or carbonization (under environment) of an organic precursor to obtain carbon inside the mesopores of silica templates.4 Alternatively, the sol-gel method has been used to assemble porous carbon flakes through a hydrolysis and condensation process using a silica source such as tetraethoxysilane [TEOS,
].5 The sol-gel approach has been widely used as a versatile method to produce inorganic–organic electrodes and electrochemical sensors for decades.3 Porous organosilicate matrices are an excellent inert support medium because of their lack of electroactivity. Therefore, much research effort has focused on immobilizing surface functional groups, chemical modifiers, or redox species within the matrices to enhance the electroactivity of the immobilized species. Electroactive silicate composites can be obtained by incorporating inorganic, organic, or biological moieties into sol-gel precursors and allowing this to gelate following film deposition.3 For example, Oskam and Searson have used micrometer-sized graphite particles to obtain 0.1 cm thick films with
conductivity with pure capacitive behavior on fluorine-doped tin oxide, copper, and steel substrates.6 Berguiga et al. have incorporated functionalized multiwalled carbon nanotubes in a sol-gel process.7
We have successfully entrapped nanosized (average diameter 55 nm with maximum diameter less than 100 nm) graphite particles in a sol-gel matrix and have obtained relatively large values of double-layer capacitance due to the combination of hydrophobicity of sol-gel and nonhydrophobicity of graphite nanoparticles as well as the enhanced surface area of the films. We also observed that the capacitance increased exponentially when the micropores in porous silica dominated the redox reaction in the double-layer capacitor. We have also been able to substantially reduce the capacitance of the films by exposure to low-power oxygen plasma. These properties could be used for many applications, including sensitive electrochemical detection of analytes and fabrication of supercapacitors.
Materials and Methods
Thin-film electrode preparation
The sol-gel precursor preparation and hydrolysis were performed sequentially in a controlled-temperature environment. For the sol-gel process, precursor solutions were prepared under acidic conditions in two steps.8 The method was described earlier by de Theije et al. ,8 where the precursor solution was diluted with ethanol to prolong the gelling time so that a thin film of this solution could be spun on a substrate. In step 1 a typical starting mixture containing TEOS [], methyltrimethoxysilane [MTMS,
], water, HCl, and anhydrous ethanol
was heated at
for 90 min. The amount of MTMS and TEOS were mixed in a
basis. A total of 0.25 mol of water were added per alkoxy group, and ethanol and HCl were added in molar ratios of 3 and
relative to the silicate precursors, thus partially hydrolyzing the silicates while preventing significant condensation. This mixture was then diluted with ethanol, water, and HCl. The final molar ratios were typically silicate (MTMS and TEOS)/ethanol/
. The prepared precursor solution was allowed to age for about 3 days for further polycondensation. After polycondensation, 0.3 g of graphite nanoparticles in powder form (55 nm mean diameter, M/s Nanostructured and Amorphous Materials, Inc.) sonicated in 5 mL ethanol for 4 h were added to the 5 mL of polycondensed sol-gel and allowed to gently mix for a short time to form a colloidal suspension. Several precleaned glass substrates were drop-cast with the sol-gel graphite mixture and the films were heated to
for 15 min for solvent removal and hardening. The graphite/sol-gel thin films were heated under a nitrogen environment to
for about an hour. The electrochemical analyses were performed on an electrochemical analyzer with all electrolytes mixed with high-purity water.
Oxygen plasma treatment
As-prepared graphite thin-film electrodes were treated with plasma for various exposure times. The plasma treatment was performed in M/s Harrick Plasma (model PDC-32G). Low radio frequency (rf) power of 6.8 W was used for
plasma treatment. Thin films with different exposure times were evaluated for their electrochemical behavior.
Surface area and pore-size distribution measurement
Nitro gen adsorption–desorption isotherms were measured using a Quantachrome Autosorb-1 automated gas sorption system and the surface areas of sol-gel samples with and without graphite nanoparticles were computed using the Brunauer–Emmett–Teller (BET) method. Pore-size distribution was determined by applying the density functional theory and Monte Carlo simulation methods using the AS1 WIN software package. The samples were degassed at for 12 h before performing the nitrogen adsorption–desorption experiments.
Contact angle measurement
We have correlated the change of contact angle using the sessile drop measurement method on the plasma-exposed film surface with the corresponding change in its capacitance value. For the oxygen plasma treated samples, contact angle measurements were performed within 1 min of treatment. The contact angle was calculated from the image of the droplet.
Fourier transform infrared spectroscopic measurements
A Nicolet-4700 Fourier transform infrared (FTIR) spectrometer was utilized for spectral measurements of the as-prepared and plasma-exposed films on low-resistivity silicon substrates in the transmission mode.
Tests and characterization
The resistivity of the films was obtained from a four-point measurement. The film thickness was determined using a profilometer and was later confirmed by scanning electron microscope (SEM). Cyclic voltammetry was performed on the films using a CHI model 800B electrochemical analyzer. The thin films, a platinum wire, and a Ag–AgCl segment (0.8 mm diam × 8 mm) were used as working, counter, and reference electrodes, respectively. Cyclic voltammograms were obtained with all three electrodes immersed within a conventional electrochemical cell. All chemicals employed were analytical reagent grade, and the measurements were taken at room temperature .
Results and Discussion
Thin-film electrode properties
The importance of using sol-gel precursor in the synthesis of carbon-based electrode materials lies in the fact that the composite material can benefit from the excellent properties of the silicate backbone. As pointed out earlier, the backbone is itself electrochemically inactive but provides an environment for electron percolation through the interconnected grains of carbon.3 The graphite/sol-gel electrodes showed a resistivity of , suggestive of the conductive nature of the carbon nanostructures. Some investigators reported that long-range hydrophobic forces in aqueous solutions can be obtained at surface contact angle,
.9–11 The as-prepared graphite/sol-gel thin-film electrodes were superhydrophobic with contact angles of
. This can be attributed to the addition of hydrophobic organofunctional monomers such as MTMS, which form hydrophobic organosilicates as well as the micrometer-scale roughness arising from the highly porous matrix structure. The nitrogen adsorption–desorption isotherms measured for both sol-gel only and sol-gel with graphite nanoparticles are shown in Fig. 1a. It is evident that the adsorption–desorption behavior is similar in both these samples. Furthermore, the shape of the isotherms indicates that the samples have bimodal pore distribution comprised of both micropores and mesopores. The surface area of the graphite/sol-gel samples measured by the BET method was
, whereas the surface area of the graphite nanoparticles and sol-gel matrix without the nanoparticles were 60 and
, respectively. The thin film contains
of porous silica binding
of graphite nanoparticles. Figure 1b shows the pore-size distribution computed for both the sol-gel and the graphite nanoparticle/sol-gel composite films. The pore-size distribution of sol-gel with graphite nanoparticles shows two peaks at 1.4 nm (micropores) and around
(mesopores) while that of sol-gel without graphite nanoparticles exhibits peaks at 1.3 and 3.3 nm. Because the graphite nanoparticles are nonporous, it is expected that the pore-size distribution and the pore volume are identical in both sol-gel samples, with and without graphite nanoparticles. Detailed pore volume analysis shows that both these samples have nearly the same pore volume of about
and a micropore volume of about
.
Figure 1. (a) Nitrogen adsorption–desorption isotherms of sol-gel samples with and without graphite nanoparticles and (b) pore-size distribution determined using density functional theory and Monte Carlo methods.
The SEM image in Fig. 2a presents the highly porous structure of the sample. Figure 2b shows mesopores in the samples having diameters less than 5 nm. The graphite nanoparticles used are spherical and have an average diameter of 55 nm, as shown in the transmission electron microscopic (TEM) image (Fig. 2c).
Figure 2. (a) SEM image of sol-gel graphite thin-film electrode showing a highly porous surface, (b) magnified SEM image of sol-gel graphite thin film showing mesopores with diameter less than 5 nm, and (c) TEM image of spherical graphite nanoparticles of 55 nm average diameter.
Capacitance analysis
The double-layer capacitance, , was measured from the current response to a triangular voltage waveform. The electrode area over which the measurements were performed was
, and the total mass of the graphite nanoparticles enclosed within this area was 0.072 mg. Different scan rates (1, 2, 5, 10, 20, 50, 100, and 200 mV/s) for the triangular waveform were applied and the change in current
was measured from the plateau region of the cyclic voltammogram (Fig. 3). The specific capacitance (F/g) was calculated from the relationship
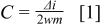
where is the scan rate and
is the mass of graphite nanoparticles in the sample. The specific capacitance per unit mass for our films with a BET surface area of
was calculated to be 36.7 F/g at a scan rate of 1 mV/s. In contrast, Oskam and Searson report a specific capacitance of 0.24 F/g for films consisting of micrometer-sized carbon particles embedded in a silica matrix. The BET surface area of their films was
.6 The 2 orders of magnitude increase in the specific capacitance of our films can be attributed to the increased specific surface area because of the use of graphite nanoparticles and the micro- as well as mesopores of our sol-gel network. In addition, we have observed that the hydrophobic nature of the sol-gel matrix plays a significant role in the high value of specific capacitance with aqueous solutions. We hypothesize that this large value of capacitance for the graphite nanoparticle/sol-gel composite results from having nonhydrophobic graphite nanoparticles embedded within a hydrophobic porous matrix (Fig. 4a). This restricts the solvated ions to tightly confined spaces close to the surface of the graphite electrode, resulting in small separations between the electrolytes and the graphite particle surface and a significant increase in the double-layer capacitance (Fig. 4b). A similar supercapacitance effect was observed by Chmiola et al.12 when the pore size of the carbon electrodes was made so small as to approach the diameter of the solvated ions. The resulting "squeezing" of the solvated ions within the pores brings them closer to the electrode surface, thereby increasing the capacitance.
Figure 3. Cyclic voltammograms of sol-gel graphite thin-film electrode on glass substrates at a scan rate of . The solid line and dotted line show the cyclic voltammetry curves of as-prepared films and
-plasma-treated films with 1 M KCl, respectively.
is taken at a point in the flat region.
Figure 4. (a) Schematic drawing of the as-prepared films show that mesopores and micropores contain many embedded graphite nanoparticles, and (b) enlarged region of the circle in (a) shows the decrease in the distance between the solvated ion center and the surface of graphite nanoparticles due to hydrophobicity.
In our case, due to the tight confinement of the electrode particles within the silica matrix, as illustrated in Fig. 4a, the solvated ions are further confined around the hydrophilic graphite electrode surface, as shown in Fig. 4b, similar to the effect observed by Chmiola et al.12 Furthermore, capacitance measurements performed for plasma-exposed films with aqueous electrolyte solutions show a much smaller value. The importance of the hydrophobic matrix in determining the high specific capacitance in aqueous electrolyte solutions is thus well understood. The specific capacitances per unit mass of as-prepared films with different thicknesses were found to be the same (data not shown), confirming that whole films (bulk) were involved in the process.
Effect of oxygen plasma exposure on the sol-gel graphite films
Low-power oxygen plasma treatment of these films has been performed to render them hydrophilic by the removal of groups from the sol-gel matrix. The films were exposed for different times and the specific capacitance per unit mass was measured immediately after each exposure. Figure 5 gives the capacitance vs oxygen plasma treatment time plots which show a dramatic drop in the capacitance from a relatively high value of 24.4 F/g for the as-prepared films to as low as 0.54 F/g at 20 mV/s after a 5 s exposure to
plasma. This behavior is consistent with our proposed model, where the hydrophobicity of the porous matrix is critical for the high specific capacitance of the material. FTIR spectra and contact angle of the films were obtained at different times of exposure to oxygen plasma to probe the mechanism for the change in specific capacitance. In Fig. 3 we compared the cyclic voltammograms of as-prepared and oxygen-plasma-treated films in 1 M KCl. The solid line and dotted line indicate the as-prepared film and oxygen-plasma-treated films, respectively. After an initial sudden drop of capacitance during the first few seconds of plasma exposure, the capacitance per unit mass begins to increase with continuing exposure, although never reaching the values obtained with the as-prepared films (Fig. 5). This can be attributed to change in surface texture with increased exposure times (Fig. 6c). The maximum capacitance observed in 95 s of exposure was calculated to be 5.88 F/g. Figure 6 shows SEM micrographs of sol-gel graphite films before and after oxygen plasma exposure. Figure 6a and 6b shows the top and cross-sectional views of as-prepared sol-gel graphite films, while Fig. 6c and 6d shows that of the plasma-exposed sample, respectively. Long-time oxygen plasma treatment created damage to the surface of the films, while no damage was observed for short-time plasma treatment. SEM cross-sectional analysis of the films suggested a substantial decrease in the film thickness, from an initial value of
(Fig. 6b) to
after the 30 min exposure (Fig. 6d).
Figure 5. Specific capacitance per unit mass of the as-prepared film and the same film exposed for different durations with low rf plasma, measured at a scan rate of
.
Figure 6. SEM micrographs of sol-gel graphite thin-film electrode: (a) top view, (b) cross section before plasma exposure, (c) top view, and (d) cross section after 30 min exposure to plasma.
Nonaqueous electrolytes have been used for further study on the hydrophobic and hydrophilic nature of electrodes. As-prepared films and oxygen-plasma-treated films with 0.1 M tetraethylammonium tetrafluoroborate/propylene carbonate showed 19.58 and 19.55 F/g at 10mV/s, respectively. Specific capacitances with nonaqueous electrolytes on as-prepared films and oxygen-plasma-treated films did not change because these are invulnerable to hydrophobicity.
FTIR spectroscopy and contact angle of the film surfaces
We have investigated the effect of oxygen plasma by performing an FTIR characterization of the films. Figure 7a shows FTIR spectra of the as-prepared films and -plasma-exposed films. The peak at
corresponds to the graphitic
stretching band, while the peak at
is attributed to Si–O stretching.13 Figure 7b presents the FTIR spectra at the region
that is attributed to the presence of
groups (where
, 2, 3, or 4) in the MTMS precursor. The area under the curve was computed before and after exposing the films to oxygen plasma for 30 min, and we observed a
decrease in the area of the
peak following oxygen plasma exposure. The as-prepared surface of the sol-gel entrapped graphite film is highly hydrophobic (contact
). The contact angle of the film after 5 s of exposure to oxygen plasma drops to approximately
(data not shown). The contact angle and FTIR measurements confirm that the film changes from superhydrophobic to hydrophilic due to the removal of
groups by oxygen plasma.
Figure 7. (a) FTIR absorbance spectra of as-prepared and - plasma-exposed sol-gel graphite thin-film electrodes. (b) Calculated absorption coefficient in the spectral region of
corresponding to the
stretching mode.
Dependence of specific capacitance on scan rate
We have observed an exponential relation between scan rates and specific capacitance which is consistent with the porous nature of our sol-gel network consisting of mesopores as well as micropores (Fig. 8). Yang et al. reported that in the case of porous carbon electrodes, mesoporous capacitance was mainly measured in fast scan rate, whereas the sum of micro- and mesoporous capacitances was measured in slow scan rate.14 At slow scan rates ions have time to move in and out of micropores, whereas this mass-transfer process is too slow to respond to fast scan rates. Also, because the micropores are smaller in size than the solvated ions, ions entering micropores in response to an electric field become partially dehydrated and are thus closer to the electrode surface than what occurs in bulk solution. This close apposition between the ion and the electrode leads to an increase in specific capacitance similar to that previously described in porous carbon electrodes.12 The dependence of capacitance on scan rate was observed in as-prepared films (Fig. 8a) and -plasma-treated films (Fig. 8b), whereas the capacitance of nonporous films such as platinum did not show scan-rate dependence (data not shown).
Figure 8. Specific capacitance (F/g) measured as a function of scan rate with (a) as-prepared electrode and (b) -plasma-treated electrode.
Conclusion
In this communication we have demonstrated that entrapment of carbon nanoparticles in sol-gel binders can be utilized as charge storage devices with desirable properties of high conductivity, high specific capacitance, and good physical stability. We have achieved high capacitance values by entrapping graphite nanoparticles in a hydrophobic porous organosilicate matrix. The restriction of the solvated ions to tightly confined spaces close to the graphite electrode surface resulted in the high capacitance values. Furthermore, plasma treatment of the as-prepared films rendered the surfaces hydrophilic and resulted in a corresponding dramatic drop in capacitance values with aqueous electrolytes. We also observed that the specific capacitance depended on scan rate, which can be attributed to the presence of micropores in the silica matrix.
Acknowledgments
This work was supported by the National Institutes of Health, grant no. NS048826 .
University of Missouri assisted in meeting the publication costs of this article.