Abstract
Wound care management and wound treatment is one of the common and challenging tasks in medicinal field. Development of cost-effective and novel nano material based drugs with wound healing properties is a promising research arena. In the present study, we report systematic comparison of the physical properties and biological activity of biogenic (ZnO-B) and chemogenic (ZnO-C) zinc oxide nanoparticles (nano ZnO). Pomegranate peel is used as a reducing agent (otherwise a waste) for the synthesis of biogenic nano ZnO. Synthesized nano ZnO were characterized using PXRD, FESEM and TEM. Wound healing potential was assessed using excision wound model for different concentrations of ZnO-B, ZnO-C and are compared with standard drug. Excision wound treated with 2% ZnO-B (95.57 ± 1.031%) exhibited highest wound closure rate compared to 2% ZnO-C (93.95 ± 1.143%) and standard drug povidone–iodine ointment (5 w v−1%) (93.29 ± 0.391%). Sections of animals treated with 2% ZnO-B showed epidermal re-epithelialization with proliferation of fibroblast and mononuclear inflammatory infiltrates, new blood vessels and significant increase in collagen formation in the dermis. When compared with the standard drug, both ZnO-B and ZnO-C showed higher MIC against all the tested strains (Staphylococcus aureus, Pseudomonas aeruginosa and Escherichia coli). ZnO-B showed higher antibacterial activity compared to ZnO-C nanoparticles. In addition to this, in silico molecular docking studies were also carried out for GSK-3β synthase and GlcN-6-P synthase. The in silico studies proves the interactions of GSK-3β synthase and GlcN-6-P synthase with ZnO. Based on the results of antibacterial activity and docking studies a probable wound healing mechanism is proposed.
Export citation and abstract BibTeX RIS
1. Introduction
In recent years, significant efforts have been made towards wound care management with an emphasis on new therapeutic approaches and technologies. Although wound healing is a natural phenomenon, persistence of chronic conditions such as diabetes mellitus, venous stasis ulcers, skin ulcers (bed sores) and other secondary complications has led to the conversion of acute wound to chronic wound making wound management a difficult task [1]. In addition to this, microorganisms prevailing at the wound site can cause severe infections by disrupting the clotting mechanism and prolonging the inflammatory phase of wound healing and angiogenesis process. These factors necessitate the requirement of wound dressings loaded with novel active components which can accelerate the wound healing process along with the prevention of microbial invasion [2].
Past decade has witnessed advanced and facile synthesis of biocompatible and antimicrobial nanomaterials with controlled morphology, increased sensitivity and specificity. This has opened up promising advancement in biomedical applications such as antibacterial coatings for implant devices, targeted drug delivery, photoablation therapy, bioimaging, hyperthermia, wound healing, etc [3, 4]. Several metal-based nanoparticles (such as Au, Ag, Cu etc.) and metal oxide nanoparticles (such as ZnO, TiO2, CeO2, Y2O3 etc.) which exhibit bacteriostatic and bactericidal activities with low in vivo toxicity have been reported as antimicrobial agents in wound healing [5–7]. Among the various nanomaterials, nano ZnO, owing to its excellent antimicrobial, anti-inflammatory, wound healing (acute as well as chronic) and angiogenic properties, is widely researched as bioactive compound for biological applications [8].
Among various physical characteristics, particle size and morphology play crucial role in controlling the physical, chemical, electronic, optical, and biological properties of nanoparticles. From numerous reports, it is evident that the antibacterial property of nanomaterials significantly varies with the size of the particles. Lu et al [9], Zhong et al [10] and Agnihotri et al [11] have observed an obvious size-dependent antibacterial and cytotoxic activity of silver nanoparticles against various bacteria and attributed it to large surface area of smaller size nanoparticles, that leads to increased interactions between particles and cells resulting in faster release of silver ions. Size dependent antimicrobial effect of palladium nanoparticles has been observed by Adams et al [12]. Palanikumar et al [13] have reported that the inhibitory efficacy of nano ZnO against infectious microorganisms like Salmonella paratyphi, K. pneumonia, Bacillus subtilis, E. aerogenes, Staphylococcus epidermidis is significantly dependent on its chosen concentration and size. The antibacterial activity of nano ZnO against S. aureus was found to increase with decrease in nanoparticles size [14].
Among various strategies of controlling the size and morphology of the nanoparticles, simplest approach is varying the precursors and reducing agents used for synthesis. Strong reducing agents like sodium borohydride (NaBH4) facilitates instant nuclei generation and results in small sized nanoparticles of narrow size distribution. On the other hand, weaker reducing agents like trisodium citrate (TSC) results in formation of relatively large size nanoparticles, having a wider size distribution [15]. In this direction, various researchers have explored the possibilities of controlling the size of metal oxide nanoparticles by various chemical and biological methods using different reducing agents [16, 17]. Chemical method of synthesis utilises chemical reducing agents and necessitates the use of stabilising agents to prevent agglomeration. On the other hand, green method of synthesis using phytoconstituents, comprising of both reducing and stabilising agents, is a cost-effective one-step procedure carried out under physiological pH, temperature and pressure [18]. Green synthesis has been recently gaining interest due to simple procedures employed, ease of raw material availability, safe precursors used, negligible generation of noxius byproducts etc. Nano ZnO have been synthesized through green fuel approach using phytoconstituents of various plants like coffee husk [19]; Trianthema portulacastrum Linn. (TP) [20]; Artocarpus Heterophyllus [21]; Calotropis gigantea [22, 23]; Polygala tenuifolia [24]; Camellia sinensis [25]; gum tragacanth [26]; Citrus aurantafolia [27]; Euphorbia tirucalli [28] etc.
Inspite of extensive reports on the biogenic synthesis and biological activity of nano ZnO have been reported [8], systematic comparative study on the influence of chemical and biological reducing agents on the physical properties and biological activity of nanoparticles has not been investigated. In this regard, present study demonstrates the impact of chemical and biological approach of synthesis on the antibacterial activity and wound healing potential of ZnO nanoparticles. The prime novelty of the work is utilization of Pomegranate peel as a reducing agent (otherwise a waste) for the synthesis of nano ZnO for the application as bioactive agent for wound healing. Systematic comparison of the physical properties and biological activity of biogenic and chemogenic ZnO is investigated. Antibacterial activity and molecular docking studies on selective enzymes are also investigated to understand the mechanistic aspects of wound healing.
2. Materials and methods
2.1. Materials
Zinc nitrate hexahydrate (Zn(NO3)2.6H2O; 99.9%), Zinc acetate dihydrate (Zn(CH3.COO)2.2H2O; 99.9%), Sodium carbonate anhydrous (Na2CO3, 99.9%) were obtained from Sisco Research Laboratories Pvt. Ltd (SRL), Mumbai. All the solutions and aqueous extract were prepared using double distilled water. Healthy fruits of Pomegranate (Punica granatum L.) were collected from local market of Bangalore, India.
2.2. Synthesis of chemogenic and biogenic ZnO nanoparticles
2.2.1. Processing of plant material
Pomegranate (Punica granatum L) fruits were gently washed with Millipore water to remove the surface dirt and debris. The arils and peel were separated; the separated peel was dried at 50 °C in hot air oven overnight and homogenized in a domestic mixer. 10 g of finely ground dried peel powder in 100 mL of double distilled water was heated for 45 min at 60 °C. The extract obtained was cooled and centrifuged at 10000 rpm for 10 min The supernatant was collected into a clean screw cap vial for further studies [29].
2.2.2. Synthesis of nano ZnO
2.2.2.1. Chemogenic synthesis of ZnO NPs
Chemogenic ZnO nanoparticles (ZnO-C) was prepared by precipitation method reported earlier [29]. In brief, 0.1 M Zinc nitrate hexahydrate was added dropwise to 0.12 M sodium carbonate solution under vigorous stirring. The precipitate formed was collected by centrifuging at 10 000 rpm for 10 min The resultant precipitate was washed with distilled water for two times and ethanol for one time. The milky white colour solids were then dried at 100 °C for 6 h and calcined at 600 °C for 2 h.
The reactions that occur during the chemogenic synthesis of ZnO NPs can be explained as follows; Synthesis of zinc oxide nanoparticles was carried out by using zinc nitrate hexahydrate and sodium carbonate as precursors. In this process, zinc carbonate is formed as intermediate product (white precipitate), which when calcinated ∼350 °C forms zinc oxide by decomposition of zinc carbonate producing CO2 [29–31]. The reaction of zinc oxide formation are shown as follows [32]:


2.2.2.2. Biogenic synthesis of ZnO NPs
For the biogenic synthesis of ZnO nanoparticles (ZnO-B), 0.2 M of zinc acetate dihydrate was dissolved in 70 mL of distilled water and stirred for few minutes. 30 ml of Pomegranate peel extract (PPE) was added to this solution and mixed homogenously at 60 °C for 40 min. Further, it was cooled to room temperature and centrifuged at 10 000 rpm for 10 min. The resultant precipitate was washed with distilled water for two times and ethanol for one time. The obtained yellowish white precipitate was dried overnight at 60 °C and calcined at 500 °C for 2 h [25].
Pomegranate peel extract is known to majorly contain punicalagin and gallic acid along with other phenolic compounds such as ellagic acid, chlorogenic acid, caffeic acid, punicalin, apigenin, quercetin, pelargonidin, cyanidin, granatin A, granatin B etc, that are proven to be involved in biosynthesis of various metal and metal oxide nanoparticles [33–35]. Negatively charged atoms present in gallic acid and punicalagin can donate electrons resulting in stabilization of the positively charged Zn2+ complex ions. Further, calcination at 500 °C leads to decomposition of Zn2+ complex ions to stable nano zinc oxide. The reaction mechanism is as follows [36]:

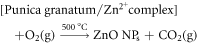
2.3. Characterization studies
Characterization studies of synthesized ZnO nanoparticles were carried out using various analytical techniques. Crystalline phases were analysed by Powder x-ray diffraction (XRD) using Rigaku Ultima IV, Japan diffractometer with Cu Kα radiation (λ = 1.541 Å).The morphology of the samples were analysed by scanning electron micrographs (SEM) using Carl Zeiss ULTRA55, Germany Field Emission Scanning Electron Microscopy (FESEM). Since ZnO is insulating, gold sputtering was done prior to electron micrography to get clear images. Transmission electron microscopy (TEM) was performed on a Jeol/JEM 2100 (accelerated voltage upto 200 kV, LaB6 filament) Transmission electron microscope.
2.4. Wound healing activity
2.4.1. Preparation of formulation
Chemogenic and biogenic ZnO nanoparticles (i.e., ZnO-C and ZnO-B) formulations of 1% and 2% were prepared using paraffin wax as ointment base to evaluate its efficacy in comparison with povidone–iodine ointment (5% w/w). Drug formulations were prepared freshly every fourth day.
2.4.2. Evaluation of wound healing activity
Excision wound model was used to evaluate the wound healing activity of synthesized Chemogenic and biogenic ZnO nanoparticles (i.e., ZnO-C and ZnO-B). Entire animal study and experimental protocol was conducted as per the Institutional Animal Ethics Committee for the purpose of control and supervision of experiments on animal guidelines, India (Approval number IAEC: 1165/bc/08/CPCSEA/17/2015). Excision of wounds were made as described by Morton and Malone [37]. Animals were anaesthetized with anesthetic ether and placed in operation table in its natural position. A square wound of about 500 mm2 was made on depilated ethanol-sterilized dorsal thoracic region of rats. After wound creation, experimental animals (i.e., Male albino Wistar rats) weighing 110 to 150 g were divided into 6 groups of 8 rats each. Out of six groups of animals, group—I received ointment base (control); Group-II were treated with a 5%w/w povidone–iodine ointment; Group-III were treated with ZnO-B ointment (1%w/w); Group-IV were treated with ZnO-B ointment (2%w/w); Group-V were treated with ZnO-C ointment (1%w/w) and Group-VI were treated with ZnO-C ointment (2%w/w).
The wound contraction was studied by tracing the raw wound area subsequently on day 1, 3, 7, 11, 14, 17 and 21. During course of excision wound model analysis, there was no death of any rats in the study groups. Scar residue, area and time of complete epithelisation were also measured.
The percentage of wound contraction was calculated as:

Where n stands for number of days of treatment (3rd, 7th, 11th, 14th, 17th and 21st day)
Results are expressed as the mean ± S.D (n = 8). Statistical significance (p) was calculated by one-way ANOVA followed by Dennett's test employing statistical software, GraphPad, InStat 3 and by comparing treated group with control. p values <0.05 mean between paired observations were considered statistically significant.
2.4.3. Histopathological analysis
Histopathology is the microscopic study of tissues for pathological alterations. This involves collection of morbid tissues from biopsy or necropsy, fixation, preparation of sections, staining and microscopic examination. In this technique on 21st day of excision wound, thin pieces of 3 to 5 mm thickness of skin fragments with scar is excised, showing gross morbid changes along with normal tissue and fixed completely in 10% formalin solution for 24–48 h. After fixation, sections were deparaffinised and stained with haematoxylin and eosin. Further, the sections were DPX mounted and observed under microscope for Congested blood vessels (CB), Mononuclear inflammatory infiltrates (MI), Proliferation of fibroblast (PB), Focal mononuclear inflammatory infiltrate (FM), Reepithelialisation (RE), Fibrocollagenous stroma (FS), Neutrophils (N), Inflammatory cells (IC), Thin walled congested vessels (TC), foreign body material (FB) and Inflammatory infiltrates (IF).
2.5. Antibacterial activity
A 3200 μg ml−1 stock solution of Ciprofloxacin was prepared by dissolving 0.32 mg of (Ciprofloxacin) in 0.1 ml of sterile acidified water (0.1 N HCl solution). Similarly, stock solutions of test samples (ZnO-C and ZnO-B) were prepared by dissolving 1.0 mg of test sample in 0.313 ml of DMSO separately. The stock solutions were diluted was serially diluted by two-fold dilution to obtain a series of working stock (WS) solutions.
One day before the initiation of experiment, the bacterial strains (Escherichia coli, Staphylococcus aureus, Bacillus subtilis and Pseudomonas aeruginosa) were picked from isolated colonies, inoculated into sterile cation adjusted Muller Hinton Broth (CAMHB) tubes and incubated overnight at 37 °C to exponential-phase. Post incubation, the cultures were identified by Gram staining and optical density is adjusted to that of a 0.5 McFarland Standard (Approximately equivalent to a bacterial suspension 1.0 × 108 CFU ml−1), and diluted to ∼1.0 × 107 CFU ml−1.
Micro dilution method was used for determination of MICs as per Clinical and Laboratory Standard Institute (CLSI) [R F Cockerill. Methods for dilution antimicrobial susceptibility tests for bacteria that grow aerobically; Approved Standard—Ninth Edition. Clinical and Laboratory Standards Institute (CLSI). 32 (2012) 1–68]. The MIC assay was performed in 96 well microtitre plates. 150 μl of CAMHB broth containing working stock solutions of test samples (ZnO-C and ZnO-B) of varying concentrations were added into each well. Ciprofloxacin was used as reference compound and DMSO served as vehicle control. Each well was inoculated with 50 μl of bacterial culture (∼1.0 × 107 CFU ml−1). The plates were incubated at 37 °C for 24 h. Post incubation, plates were visually examined for turbidity and the optical density was read at 630 nm in a Spectrophotometer. The assay was done in triplicates and the obtained results were statistically analysed using ANOVA software.
2.6. Molecular docking studies
For in silico wound healing and antimicrobial activity studies, Glycogen synthase kinase-3β (GSK-3β) and glucosamine-6-phosphate synthase (GlcN-6-p synthase) are considered as target enzymes respectively. The structure of the target enzymes was obtained from Protein Data Bank (PDB ID; 1Q5K and PDB ID; 2VF5). Wurtzite structure of Zinc oxide was obtained as PDB file using Vesta software. Active pockets for proteins were acquired from CASTp server [38]. Intermediator steps, such as energy minimization, protein and ligands preparation and grid box creation were completed using Graphical User Interface program AutoDock Tools (ADT). AutoDock/Vina was employed for docking using protein and ligand information along with grid box properties in the configuration file. AutoDock/Vina employs iterated local search global optimizer [39, 40]. During the docking procedure, both the protein and ligands are considered as rigid. After the successful completion of the docking runs, different conformations of the ligands known as binding modes were obtained with their respective binding affinity and the stable one which happens to be the one with the lowest binding energy was picked and aligned with receptor structure for further analysis [41].
3. Results and discussion
3.1. Powder X-ray diffraction (PXRD) analysis
XRD patterns of the products obtained by chemogenic and biogenic methods of synthesis are shown in figures 1(a) and (b) respectively. The two products are labelled as ZnO-C and ZnO-B respectively for chemogenic and biogenic derived products henceforth. All the planes of the diffraction pattern matches with wurtzite structure and can be readily indexed to standard data (JCPDS70-0134). Keen observation of the diffraction pattern reveals that ZnO-B shows relatively broad peaks compared to ZnO-C. Otherwise, diffraction peak positions in both the samples are found to be similar with no significant variation. In order to evaluate the influence of synthesis method on crystallite size of the products, the average crystallite size (D) of ZnO-C and ZnO-B are calculated by x-ray peak broadening method using Full width half maximum of the diffraction peaks by Scherrer's formula [42]. From Scherrer's method the crystallite size of the products ZnO-C and ZnO-B are found to be 19 and 13 nm respectively. Further, it is also interesting to note that the peak intensity of the ZnO-C is found to be significantly higher compared to ZnO-B. This suggests that by varying the method of synthesis, crystallinity of the samples are also varied. The lower crystallinity and crystallite size of ZnO-B can be attributed to the lower synthesis temperature for biogenic method compared to chemogenic process. Lower crystallite size can also be explained based on the phytoconstituents acting as capping agents which may restrict the crystallite growth [43].
Figure 1. Powder x-ray Diffraction pattern of (a) ZnO–C and (b) ZnO-B.
Download figure:
Standard image High-resolution imageVarious parameters such as peak shift, peak broadening, peak asymmetry etc of the XRD pattern can be used to evaluate the crystal structure of the material under investigation using Rietveld refinement method [44, 45]. In the present study Rietveld refinement for the experimentally obtained XRD data are carried out in order to evaluate the influence of method of synthesis on structural parameters. The output of the refinement carried out for ZnO-C and ZnO-B using FULLPROF program is shown in figure 2. Using the pseudo-Voigt function various parameters are fitted with experimental data set [46]. Integrated intensity of the diffraction peaks as a function of structural parameters was considered and following Marquardst least squares procedure refinement was carried out. Refinement was performed to minimise the difference between calculated and experiment powder diffraction patterns. The final refinement analysis shows that the experimental and calculated PXRD patterns obtained by the Rietveld refinement are in good agreement with each other. The model crystal structure generated using Rietveld refinement parameters finally obtained is shown in figure 3. The refined parameters, reliable factors calculated are summarized in table 1.
Figure 2. Rietveld refinement of (a) ZnO-C and (b) ZnO-B. The patterns represented in black and red correspond to experimentally observed and calculated peak profiles, respectively. Green lines represent the background and the blue line is the difference between experimentally observed and calculated peak profiles.
Download figure:
Standard image High-resolution imageFigure 3. Packing diagram of wurtzite ZnO, obtained using rietveld parameters.
Download figure:
Standard image High-resolution imageTable 1. Rietveld refined structural parameters of Wurtzite ZnO nanoparticles prepared using Biogenic and Chemogenic methods.
Parameters | Compound | |
---|---|---|
ZnO-B | ZnO-C | |
Crystal system | Wurtzite | Wurtzite |
Space group | P 63 mc | P 63 mc |
a = 3.248 Å | a = 3.251 Å | |
Lattice parameter | ||
c = 5.20 Å | c = 5.210 Å | |
Cell Volume | 47.56(3) (Å)3 | 47.76(1) (Å)3 |
Zn | ||
X | 0.333(3) | 0.333(3) |
Y | 0.666(6) | 0.666(6) |
Z | 0.000 | 0.000 |
O1 | ||
X | 0.333(2) | 0.3975(2) |
Y | 0.666(2) | 0.1515(2) |
Z | 0.3831(2) | 0.3819(2) |
R-factors | ||
Rp | 7.78 | 5.65 |
Rwp | 9.35 | 7.61 |
RBragg | 2.19 | 1.98 |
RF | 1.70 | 1.23 |
χ2 | 1.65 | 7.74 |
3.2. Scanning electron microscopy (SEM)
The scanning electron micrographs of ZnO-B and ZnO-C are shown in figures 4(a)–(d) respectively. SEM micrographs of ZnO-B exhibits porous and fluffy morphology. Very fine and near spherical particles forming inter connected foamy structures are found to be highly agglomerated. Higher magnification SEM images (figure 4(b)) of ZnO-B shows that agglomerated particles are found to form clusters of various sizes. On the other hand, micrographs of ZnO-C are found to have larger particle agglomerates with more number of voids in their structure. Careful observation of micrographs also reveals the surface structure of ZnO-C also found to be rich with numerous pores and voids. However, unlike ZnO-B, individual particles could not be differentiated in ZnO-C due to high agglomeration nature of particles. The likely reason for higher agglomeration in ZnO-C may be due to higher calcination temperature required in this process compared to ZnO-B. The difference in surface morphology between ZnO-B and ZnO-C may be attributed to the difference in the mechanism of particle formation and the variation in synthesis temperature of the two methods. It is observed that biogenic synthesis using plant extracts is a controlled equilibrium process resulting in energetically favourable spherical shape particles [43]. Sudhasree et al [2014] have reported that biogenic nickel nanoparticles synthesised using extract of Desmodium gangeticum were of reduced size and monodispersed when compared to chemogenic nickel nanoparticles [47].
Figure 4. Scanning electron microscopic images of (a), (b) ZnO-C and (c), (d) ZnO-B.
Download figure:
Standard image High-resolution imageThe difference in the morphological features and extent of agglomerations in ZnO-C and ZnO-B can be explained as follows; Phyto-extracts have been extensively used for synthesis of various nanoparticles and these extracts contain phytoconstituents such as polyphenols, polysaccharides, proteins, terpenoids, flavones, amino acids, vitamins and alkaloids etc which act as both reducing and capping agents leading to the formation of nanoparticles. Phenols and flavonoids in particular have unique property to cap nanoparticles, thus preventing agglomeration of nanoparticles [48, 49]. Further, hydroxyl and carboxyl groups of phenolic compounds have strong affinity to bind to metals and metal ions leading to reduced agglomeration [50].
Pomegranate peel extract is known to majorly contain punicalagin and gallic acid along with other phenolic compounds such as ellagic acid, chlorogenic acid, caffeic acid, punicalin, apigenin, quercetin, pelargonidin, cyanidin, granatin A, granatin B etc, that act as act as reducing and stabilizing agents in bio-mediated synthesis of various metal and metal oxide nanoparticles [33–35]. Recently, Sukri et al [36] reported the role of gallic acid and punicalagin as stabilizing agents for the synthesis of ZnO nanoparticles. On the other hand Farrokhnia et al [51] showed that the hydroxyl groups of gallic acid can act as capping agents during the synthesis of AgNPs. Further, Wang et al [52] also reported that use of gallic acid helps in stabilization and prevention of agglomeration of gold nanoparticles. Therefore, from these literature reports we infer that, the reason for lesser agglomeration in ZnO-B compared to ZnO-C is due to the presence of phytoconstituents in general, gallic acid and punicalagin in particular that act as capping agents.
3.3. Transmission electron microscopy (TEM)
Figures 5(a), (b) and (d), (e) shows Transmission electron micrographs of ZnO-B and ZnO-C respectively. TEM micrographs of ZnO-B shows particles are nearly spherical and are uniform in size. However, particles are found to be agglomerated with uniform distribution of particles. The particles in ZnO-B are found to be in the range of 10–20 nm. However, from ZnO-C TEM images, it can be seen that the particles are uneven in size and the shape of the particles are also not uniform. The average sizes of the particles are found to be in the range of 60–80 nm and a few particles are found to be >100 nm. The bigger particle size of ZnO-C compared to ZnO-B might be due to the difference in the calcination temperature and the mechanism of particle formation. HRTEM images of ZnO-B and ZnO-C (figures 5(c) and (f) respectively) shows clear well resolved lattice fringes showing highly crystalline nature of the products. The interplanar distance from HRTEM images is found to be 0.27 nm corresponding to (002) plane in ZnO [53].
Figure 5. Transmission electron microscopic images (a), (b) ZnO-B (d), (e) ZnO-C. HRTEM of (c) ZnO-B and (f) ZnO-C.
Download figure:
Standard image High-resolution image3.4. Evaluation of wound healing activity
Wound healing effects of ZnO-C and ZnO-B formulations of 1% and 2% along with control (only ointment base) and standard group (povidone–iodine ointment (5%)) were evaluated by excision wound model (figure 6). The apparent changes in wound contraction area are tabulated in table 2. On the 17th day significant wound closure rate was observed for the test groups compared to control group. 2% ZnO-B (89.05 ± 0.47%) exhibited highest wound closure rate compared to standard (88.08 ± 0.72%) and control group (75.76 ± 2.149%). On the 21st day, 2% ZnO-B exhibited 95.57 ± 1.031% wound contraction compared to standard ointment (93.29 ± 0.391%) and control (81.54 ± 0.67%). Whereas 1% ZnO-C (92.84 ± 1.091%), 2% ZnO-C– (93.95 ± 1.143%), 1% ZnO-B—(93.29 ± 0.391%) exhibited relatively less wound closure rate compared to 2% ZnO-B—(95.57 ± 1.031%). The results obtained suggest that 2% ZnO-B had the highest wound healing property.
Figure 6. Wound healing process in the excision wound model at 7th, 14th and 21st day in presence of control, standard drug, 2%ZnO-C and 2% Zno-B.
Download figure:
Standard image High-resolution imageTable 2. Effect of ZnO-C and ZnO-B on excision wound contraction .
Number of days | Control | Standard (Povidone-iodine) | 1% ZnO-B | 2% ZnO-B | 1% ZnO-C | 2% ZnO-C |
---|---|---|---|---|---|---|
3rd day | 27.8 ± 1.316 | 36.1 ± 4.416 | 24.21 ± 2.086 | 31.58 ± 2.412 | 30.36 ± 3.165 | 35.01 ± 3.788 |
7th day | 54.74 ± 1.483 | 55.92 ± 1.572 | 62.58 ± 2.163 | 56.19 ± 3.727 | 65.79 ± 1.835** | 58.64 ± 1.533 |
11th day | 60.11 ± 4.42 | 70.29 ± 2.71 | 71.34 ± 2.01* | 69.22 ± 2.518 ns | 73 ± 2.017* | 68.53 ± 1.872 |
14th day | 68.4 ± 2.746 | 80.83 ± 1.618** | 81.34 ± 2.077** | 78.28 ± 2.121* | 81.52 ± 2.393** | 83.27 ± 1.261*** |
17th day | 75.76 ± 2.149 | 88.08 ± 0.72*** | 83.29 ± 2.513* | 89.05 ± 0.47*** | 86.72 ± 2.647** | 87.4 ± 1.204** |
21st day | 81.54 ± 0.67 | 91.8 ± 0.597*** | 93.29 ± 0.391*** | 95.57 ± 1.031*** | 92.84 ± 1.091*** | 93.95 ± 1.143*** |
Values are expressed as the mean ± S.D (n = 8); Statistical significance (p) was calculated by one way ANOVA followed by Dennett's test and by comparing treated group with control - *P < 0.001, **P < 0.01, ***P < 0.05.
Sudheesh Kumar et al [54] have reported the wound healing potential of β-Chitin hydrogel/ chemogenic nano ZnO composite bandage. ZnO was prepared by chemogenic precipitation method. Composite bandage treated wounds showed 70% and 85% wound closure after 14 days and 21 days respectively. Arshad et al [55] have reported the wound healing activity of chemogenic nano ZnO embedded thiolated chitosan-alginate bandage. Wound closure of ∼80% was observed after 12 days of treatment and complete healing was reported after 28 days. Yadav et al [20] have reported the wound healing activity of Trianthema portulacastrum Linn. plant extract mediated biogenic zinc oxide nanoparticles using excision wound model. The percentage wound closure rate of ZnO on 6th day was found to be ∼27%. Significant wound closure rate was observed only form 9th day onwards. Nano ZnO treated wounds showed ∼67%, ∼80% and ∼85% wound closure after 12, 15 and 17 days respectively.
In the present study, significant wound closure rate was observed from 7th day itself for both biogenic and chemogenic ZnO treated wounds. Wound healing activity of ZnO-B was comparable to that of ZnO-C. Percentage wound closure was ∼80%, ∼85% and ∼93% after 14, 17 and 21days for both ZnO-B and ZnO-C treated wounds. The obtained results indicate that the activity of synthesized biogenic and chemogenic ZnO nanoparticles are higher than that reported for chemogenic ZnO and comparable to that of biogenic ZnO reported in literature. Absence of impurities and smaller size of synthesized zinc oxide nanoparticles attributes to significant wound healing activities observed in the present study.
3.5. Histopathological studies
Histopathological examination of excision wound tissue was performed on 21st day and the micrographs are shown in figure 7. The micrograph revealed that animals treated with only ointment base (i.e., control group) showed normal skin epidermis. Dermis showed proliferation of fibroblasts and mononuclear inflammatory infiltrates with thin walled congested vessels indicating incomplete healing of wounds (figure 7(a)). Sections of animals treated with standard povidine iodine exhibited complete re-epithelialization and focal mild mononuclear inflammatory infiltrates with thin walled congested vessels ((figure 7(b)). Histopathological section of animals treated with 2% ZnO-C also revealed mild mononuclear inflammatory infiltrates, complete re-epithelialization with proliferation of fibroblasts and thin-walled vessels (figure 7(c)). On the other hand, sections of animals treated with 2% ZnO-B showed epidermal re-epithelialization with proliferation of fibroblast and mononuclear inflammatory infiltrates, new blood vessels and significant increase in collagen formation in the dermis (figure 7(d)) [56].
Figure 7. Histological dermal sections of wound area stained with haematoxylin – eosin (a) control (b) standard (c) 2 % ZnO-C and (d) 2% ZnO-B.
Download figure:
Standard image High-resolution imageSimilar histopathological observations have been reported for nano ZnO treated wounds. Yadav et al [20] observed enhanced collagen formation with less inflammatory cells, re-epithelialization and keratinization for biogenic ZnO treated wounds compared with control and standard. Arshad et al [55] have reported complete re-epithelialization and intact epidermis of nano ZnO treated wounds. Topical application of biogenic Ag nanoparticles showed creation of fibroblast rich granulation tissue under the wounds with complete re- epithelialization [57].
Several mechanisms have been proposed for the wound healing activity of nano ZnO like migration of keratinocytes towards wound area by the released Zn2+ ions inducing epithelialization of wounds [54, 58]; reduction in oxidative stress and inflammatory reactions resulting in quick formation and deposition of collagen fibers, tissue granulation and reconstruction of epithelial lining [20]; anti-inflammatory effect due to inhibition of protein [20]. In the present case, wound healing activity of ZnO nanoparticles might be due to the antibacterial activity coupled with interaction with specific enzymes responsible for wound healing activity. In order to prove this antibacterial activity and molecular docking studies are performed and the results are discussed in the following sections.
3.6. Antibacterial activity
Antibacterial activity of ZnO-B and ZnO-C nanoparticles were evaluated against 3 bacterial strains (i.e., Staphylococcus aureus, Pseudomonas aeruginosa and Escherichia coli). Minimum Inhibition Concentration (MIC) for standard drug (i.e., Ciprofloxacin) against Staphylococcus aureus, Pseudomonas aeruginosa and Escherichia coli was found to be 8 μg ml−1, 8 μg ml−1 and 4 μg ml−1 respectively. From MIC values and comparing with CSLI standards, it can be inferred that bacterial strains are moderately resistant (figure 8) [59]. The MIC of ZnO-B on Staphylococcus aureus, Pseudomonas aeruginosa and Escherichia coli was 50 μg ml−1, 60 μg ml−1, and 30 μg ml−1 respectively. On the other hand ZnO-C showed MIC values of 60 μg ml−1, 80 μg ml−1 and 50 μg ml−1 respectively for Staphylococcus aureus, Pseudomonas aeruginosa and Escherichia coli. Compared to the standard drug, ZnO-B and ZnO-C showed higher MIC against all the tested strains. However, it is worth noticing that ZnO-B nanoparticles showed higher antibacterial activity compared to ZnO-C nanoparticles. Higher antibacterial activity of ZnO-B nanoparticles compared to ZnO-C can be attributed to smaller size of ZnO-B compared to ZnO-C. Smaller size nanoparticles can penetrate the bacterial cell membrane easily and cause membrane leakage and cell death. Similar observations are also reported for nickel nano particles by Sudhasree et al [47]. Where authors reported higher bacterial activity of biogenic derived Ni nanoparticles compared to chemically synthesized nickel nanoparticles. Further, it should also be noted that relatively higher antibacterial activity was observed against Gram negative strains than Gram positive strains. This is due to the fact that Gram negative bacteria exhibits less resistance to nanoparticle mediated toxicity on cell membrane interaction, because of thin peptidoglycan layer compared to Gram positive bacteria [60]. Antibacterial activity of ZnO-B and ZnO-C nanoparticles may be for the following reasons (i) interaction of nano ZnO with bacterial cells leading to ROS generation and ROS causes membrane leakage of proteins and nucleic acids by enhancing lipid peroxidation on membrane [61]. (ii) Zn2+ ions released from the nanoparticles might also damage the cell membrane and interact with intracellular components destructing bacterial cell integrity [62, 63]. Similar results and various other pathways of antibacterial activity/ mechanism and wound healing are also reported by various research groups [64–66].
Figure 8. Histogram of wound healing percentage with respect to time.
Download figure:
Standard image High-resolution image3.7. Molecular docking studies
Molecular docking can predict the affinity and activity of small molecule drug to their protein targets. GSK-3β is a widely expressed serine/threonine kinase encoded by 2 genes, GSK3A and GSK3B. GSK-3β controls the progression of wound healing and fibrosis by modulating ET-1 levels suggesting that targeting the GSK-3β pathway or ET-1 may be of benefit in controlling tissue repair and fibrogenic responses in vivo [67].
Molecular docking comparision of Zinc oxide with standard drug Povidone iodine against the GSK-3β protein, a wound healing biomarker, is presented in figure 9 and table 3. Povidone iodine exhibited a binding affinity of −5.1 Kcal mol−1. Formation of H–bond was absent and seven residues were involved in hydrophobic interactions. On the other hand, the binding site of ZnO on GSK-3β includes five residues involved in the hydrogen bond and five residues involved in hydrophobic interactions. ZnO exhibited binding affinity of −5.6 Kcal mol−1 with 7 H–bonds and hydrophobic interactions with Leu227, Gly230, Ile228, Tyr216 and Ser215. The strong binding is due to the existence of seven hydrogen bonds with three of them having bond length <3.00 Å. As evident from zero binding site similarity observed, it can be concluded that ZnO docked at completely different sites as compared to Povidone iodine. Overall analysis of the comparative insilico studies indicates that, ZnO with higher binding energy, protein-ligand complex stabilized by multiple H–bonds and good hydrophobic interactions with amino acids can be considered as effective compound for GSK inhibition.
Figure 9. A—2D Ligplot analysis and docking results showing crystal structure of GSK-3β, ligand Povidone iodine (Standard drug) and B - 2D Ligplot analysis and docking results showing crystal structure of GSK-3β with the ligand ZnO.
Download figure:
Standard image High-resolution imageTable 3. Molecular docking of ligands with GSK-3β.
Ligands | Target Protein | Affinity (kcal/mol) | H-BONDS | H-Bond Length (Å) | H-Bond With | Hydrophobic Interactions |
---|---|---|---|---|---|---|
Povidone iodine | GSK-3β | −6.4 | 1 | 2.93 | Arg180 | Ile217, Gly202, Val263, Phe291, Tyr216, Lys292. Ser203 |
ZnO | GSK-3β | −5.3 | 6 | 2.84 | Asp200 | Lys183, Gly202, Phe67, Ser203 |
2.92 | Ser66 | |||||
2.91, 2.76 | Asp264 | |||||
3.02 | Ser261 | |||||
2.72 | Asp181 |
In addition to the docking studies of GSK-3β protein, in silico molecular docking was also performed for glucosamine-6-phosphate synthase (GlcN-6-P synthase). The enzyme GlcN-6-P synthase is involved in the formation of N-acetyl Glucosamine, which is an essential building block of bacterial and fungal cell walls [68]. Hence, GlcN-6-P can be a promising target for antibacterial and antifungal drug discovery. Ciprofloxacin has been reported to be a good inhibitor of GlcN-6-P synthase [69]. Considering GlcN-6-P synthase as the target receptor, comparative molecular docking studies of Zinc oxide with standard drug Ciprofloxacin was performed and the results are presented in figure 10 and table 4. Standard antibiotic Ciprofloxacin exhibited binding affinity of −7.0 Kcal mol−1 with 2 H–bonds (Thr302, Ser401) and hydrophobic interactions with Ala400, Gln348, Ser303, Cys300, Asb354, Val605, Thr352 and Val399. On the other hand, ZnO exhibited binding affinity of −4.9 Kcal mol−1 with 8 H–bonds and hydrophobic interactions with Ser303, Thr302, Gly301 and Ser604. The binding site of ZnO on GlcN-6-P synthase includes five residues involved in the hydrogen bond as against only two residues in case of Ciprofloxacin. Overall analysis of the in silico studies indicates that ZnO with higher protein-ligand complex stabilized by multiple H–bonds and good hydrophobic interactions can be considered more effective than the standard drug Ciprofloxacin for inhibition of GlcN-6-P synthase. The obtained results correlate to the observed antibacterial activity. It can be concluded that ZnO exhibits remarkable antibacterial activity through the inhibition of GlcN-6- P synthase.
Figure 10. A—2D Ligplot analysis and docking results showing crystal structure of GlcN-6-P synthase, ligand ciproflaxacin (Standard drug), B—2D Ligplot analysis and docking results showing crystal structure of GlcN-6-P synthase with the ligands ZnO.
Download figure:
Standard image High-resolution imageTable 4. Molecular docking of ligands with GlcN-6-P
Ligands | Target Protein | Affinity (kcal/mol) | H-BONDS | H-Bond Length (Å) | H-Bond With | Hydrophobic Interactions |
---|---|---|---|---|---|---|
Ciprofloxacin | GlcN-6-P synthase | −7.0 | 2 | 2.99 | Thr302 | Ala400, Gln348, Ser303, Cys300, Asp354, Val605, Thr352, Val399 |
2.80 | Ser401 | |||||
ZnO | GlcN-6-P synthase | −4.9 | 8 | 3.19 | Cys300 | Ser303, Thr302, Gly301, Ser604 |
3.05, 5.20, 3.16 | Thr352 | |||||
2.73 | Ser347 | |||||
2.88, 3.19 | Ser349 | |||||
3.09 | Gln348 |
4. Conclusion
In summary, nano ZnO was successfully prepared by chemical and biological methods using chemical reducing agent (ZnO-C) and pomegranate peel extract (ZnO-B) respectively. SEM and TEM analysis showed smaller size and uniform distribution of ZnO-B particles (10–20 nm) compared to ZnO-C (60–80 nm). Wound healing activity of ZnO-B and ZnO-C were evaluated using excision wound model on male albino Wistar rats. On the 21st day, 2% ZnO-B (95.57 ± 1.031%) treated wounds exhibited significant wound contraction compared to wounds treated with 1% ZnO-B (93.29 ± 0.391%), 1% ZnO-C (92.84 ± 1.091%), 2% ZnO-C (93.95 ± 1.143%), standard (93.29 ± 0.391%) and control group (81.54 ± 0.67%). Further, antibacterial activity results confirmed noticeable activity on both Gram negative and Gram positive bacteria. MIC on Staphylococcus aureus, Pseudomonas aeruginosa and Escherichia coli of ZnO-B (50 μg ml−1, 60 μg ml−1, and 30 μg ml−1 respectively) and ZnO-C (60 μg ml−1, 80 μg ml−1 and 50 μg ml−1 respectively) indicate enhanced antibacterial effect of ZnO-B compared to ZnO-C, due to the smaller size of the biogenically derived ZnO. In addition to this, in silico molecular docking studies revealed that nano ZnO with higher binding energy (−5.6 Kcal mol−1), protein-ligand complex stabilized by multiple H–bonds (7 H–bonds) and good hydrophobic interactions with amino acids effectively inhibited GSK-3β synthase thereby enhancing the wound healing. ZnO docked at completely different sites as compared to Povidone iodine. In silico molecular docking performed for glucosamine-6-phosphate synthase (GlcN-6-P synthase) indicated binding affinity of −4.9 Kcal mol−1 with 8 H–bonds and hydrophobic interactions for nano ZnO. Inhibition of GlcN-6-P synthase, an essential building block of bacterial and fungal cell walls, by nano ZnO was comparable to standard drug Ciprofloxacin contributing to its antibacterial activity. In conclusion ZnO exhibits remarkable wound healing and antibacterial activity through inhibition of GSK-3β and GlcN-6-P synthase respectively.