Abstract
The critical demand for eco-friendly, renewable, and safe energy resources is an essential issue encountered in the contemporary world. The catalytic transesterification of plant oils into biodiesel was assessed as promising a technique for providing new forms of clean and safe fuel. Natural clinoptilolite was doped with Na+ ions by green chemical reactions between sodium nitrite and green tea extract, producing a novel modified structure (Na+/Clino). The Na+/Clino product had an enhanced total basicity (6.41 mmol OH/g), ion exchange capacity (387 meq/100 g), and surface area (312.7 m2 g−1), which qualified it to be used as a potential basic catalyst for the transesterification of palm oil. Transesterification tests were statistically assessed using a response surface methodology and a central composite design. Considering the effect of how the significant factors interact with each other, the synthetic Na+/Clino achieved a 96.4% experimental biodiesel yield after 70 min at 100 °C in the presence of 2.75 wt% catalyst loading and a 12.5:1 methanol-to-palm-oil ratio. Based on the optimization function of the statistical model, the performance of Na+/Clino can theoretically be enhanced to increase the yield to 98.2% by expanding the test time to 85 min and the loading value to 3 wt%. The product yielded by the Na+/ClinO process is of adequate technical properties, considering the international levels for standard biodiesel (EN 14214 and ASTM D-6751). Finally, the prepared green Na+ doped clinoptilolite had excellent recyclability as a heterogeneous basic catalyst and displayed higher efficiency than several species of previously studied heterogeneous and homogenous catalysts.
Export citation and abstract BibTeX RIS
1. Introduction
Globally, the urgent need for sustainable and clean energy resources is one of the main challenges to the progress of the contemporary world [1, 2]. Additionally, the unguided consumption of traditional fuels resulted in numerous toxic emissions such as CO, CO2, BTEX compounds, sulfur, and nitrogen oxides [3, 4]. Therefore, the need for renewable energy resources with low production costs, low toxic emissions, and promising technical properties has shaped the direction of research in recent times [5, 6].
Green biofuels and biodiesel made from edible and nonedible plant oils, biomass extracts, vegetable oils, animal fats, and algae extracts have been presented as potential alternative products to fossil-based fuels [7–9]. From an environmental viewpoint, biodiesel produced from the previously mentioned green resources has excellent biodegradable properties, low toxic emissions, and low CO2 production [10, 11]. Technically, it has appropriate viscosity, promising lubricity, a significant cetane number, and a high flash point. Such properties signify the qualifications of biodiesel products that can be used as engine fuel or as essential components to be blended with petrodiesel [12–14].
Chemically, biodiesel is a name for types of fatty-acid alkyl ester that can essentially be extracted by transesterification reactions of plant oils using alcohol and a suitable catalyst (homogenous or heterogeneous) to induce the process [15, 16]. Most of the presented studies have demonstrated significant results for homogenous catalytic reactions, but unfortunately, such reactions are subject to several environmental and economic limitations [16, 17]. The chemicals used are very expensive and have corrosive effects, difficult separation processes, no recyclability, and a high saponification effect [3]. Recently, transesterification reactions based on heterogeneous catalysts instead of homogenous catalysts have been widely investigated, to avoid the previously reported economic and environmental drawbacks [18]. The heterogeneous forms of the catalysts used in such reactions have been recommended as environmentally safe products that have stable chemical structures, low leaching ability, and no toxic byproducts [19, 20]. Additionally, the heterogeneous forms have significant technical properties, such as low fabrication costs, simple separation methods, and high recyclability value [19, 20].
Fabrication costs, the availability of materials, catalytic activity, dispersion properties, and safety values are essential considerations during the production of heterogeneous catalysts [1]. Therefore, modifications of natural minerals and rocks by alkaline ions as basic groups (K+, Ca+2, Na+, Mg+2), or by acidic groups, have been introduced as promising techniques to produce potential catalysts meeting the requirements for low cost, high natural availability, high activity, and significant safety [1, 3]. Among the extensively examined minerals, the natural zeolite minerals (mordenite, clinoptilolite, and heulandite) showed favorable catalytic influence in transesterification reactions, especially in modified forms [21–23].
Clinoptilolite is an abundant species of natural zeolite with a microporous silicate structure, which exhibits high mechanical strength, surface area, thermal stability, and ion exchange capacity, and a safe chemical composition [24, 25]. Unfortunately, the catalytic activity and behavior of pure, as well as treated, clinoptilolite were not studied sufficiently in the transesterification of both edible and nonedible oils. Additionally, the reported enhancements in the physicochemical properties of such silicate minerals based on different modification techniques (alkali, acids, and metal oxides) reflect the potential of modified clinoptilolite to be used effectively in the transesterification process [22, 26, 27].
Recently, the application of the green extracts of plants, leaves, and even enzymes as reducing and stabilizing agents in green modification reactions and the synthesis of nano metal oxides was strongly recommended as a safe and low-cost technique [28]. Synthetic or modified materials made by such methods have excellent environmental properties, safety, biodegradability, surface area, surface reactivity, and enhanced catalytic performance [29]. It was expected that the possible modifications of clinoptilolite mineral by alkalis using green treatment techniques would result in a novel modified form of clinoptilolite that was expected to have high surface reactivity, catalytic activity, and more environmental properties [1].
Therefore, this study aimed to develop green Na+ doped clinoptilolite (Na+/Clino) by green methods in the presence of green tea extract. The synthetic Na+/Clino was characterized by different analytical techniques as a green and eco-friendly basic catalyst in the transesterification of palm oil. The catalytic performance of Na+/Clino and its transesterification behavior were investigated based on the central composite rotatable design of the response surface methodology, taking into account the essential variables. Also, the predicted mechanism was investigated and is presented in this study.
2. Experimental work
2.1. Materials
Mineral clinoptilolite with a chemical composition of SiO2 (68.39%), Al2O3 (11.52%), Fe2O3 (2.676%), Na2O (0.38%), CaO (1.65%), MgO (0.483%), K2O (4.06%), and L.O.I. (Loss of ignition) (10.99%) was used as a precursor in the modification reactions. Sodium nitrite (>97%, Aldrich) and commercial leaves of green tea were used as reagents in the alkali green reactions. Commercial palm oil and methanol (Sigma Aldrich, 99.8%) were incorporated during the transesterification reactions.
2.2. The synthesis of green Na+ doped clinoptilolite (Na+/Clino)
Prior to the modification process, the raw clinoptilolite fragments were ground and mechanically activated using a ball mill for 8 h according to the results reported by Shaban et al [30]. The ground product (3 g) was mixed with 50 ml of distilled water containing 2.5 g of NaNO2 and the mixture was stirred at 500 rpm in the presence of sonication waves (240 W) for 90 min at pH 8 and 25 °C. Subsequently, an extract of green tea leaves (50 ml), previously prepared by direct boiling of the leaves for 5 min, was suddenly mixed with the clinoptilolite/NaNO2 and stirred for about 120 min, followed by a sonication treatment for an additional 120 min at the same pH and temperature. Then, the resulting mixture (Clinoptilolite/NaNO2/green extract) was left at room conditions for 24 h to ensure effective interaction between the three components of the system. The modified solid particles were then separated from the solution, washed extensively with distilled water for 10 min, dried for 12 h at 70 °C, and labeled as Na+/Clino for further characterization and applications.
2.3. Characterization
An x-ray diffractometer of Cu-Kα radiation (PANalytical) was used to study the crystallinity of the clinoptilolite as well as the Na+/Clino within a measurement range of 5° to 70° and with a 5°/min fixed scanning speed. The morphologies of both the raw and modified samples were addressed using a scanning electron microscope (Gemini, Zeiss-Ultra 55) with an accelerating voltage of 30 kV. The elemental composition and the functional chemical groups were studied using energy-dispersive x-rays (EDX) and a Fourier transform infrared spectrometer (FTIR−8400S), respectively. The surface area and porous properties were measured using a surface area analyzer (Beckman Coulter SA3100). The total basicity of clinoptilolite and Na+/Clino was determined based on the method reported by Abukhadra and Sayed [31]. The clinoptilolite and Na+/Clino particles were immersed in a diluted HCl solution (50 ml acid +20 ml water) for 24 h. Then, 25 ml of the HCl solution was titrated using a NaOH solution of 0.1 M in the presence of phenolphthalein. The ion exchange capacities of clinoptilolite and Na+/Clino were obtained by the BaCl2 method [32].
2.4. Transesterification system
All the experiments in this study were performed in a 150 ml Teflon-lined stainless steel autoclave as a reactor. The mixing process and the controlled heating of the reactor were performed using a digital magnetic stirrer attached to a hot plate. The main parameters inspected during the transesterification experiments of palm oil over a Na+/Clino catalyst were the experimental time (min), the methanol ratio, the Na+/Clino loading (wt%), and the temperature (°C). The conditions of the experiments were adjusted based on the values suggested by the central composite statistical design (CCD) based on the response surface methodology (RSM) using Design-Expert software (Version 6.0.5). The selection of the lower and upper points of the parameters studied was performed by considering a series of random primary tests involving the separate effect of each parameter at different values, considering the lower limits at biodiesel yields of 50%. The selected lower and upper values of the parameters are presented as the actual and coded values in table S1 (available online at stacks.iop.org/NANO/32/155702/mmedia).
The main experimental procedures firstly involved the filtration of the palm oil and the heating of the studied sample (40 g) for 10 min at about 70 °C as an essential step to purify the oil from the solid suspension as well as water molecules. The Na+/Clino was then mixed with the palm oil sample at a certain loading and left to be stirred for an additional 10 min. Subsequently, the temperature was regularly upgraded to the values suggested by the statistical design, followed by the addition of the alcohol to the palm oil at the suggested molar ratio. Finally, the reactants were stirred with each other for certain periods according to the values suggested for the design.
After certain intervals, determined by the design, the Na+/Clino particles were separated from the liquid phases by centrifugation. The liquid portions of the experiments were then carefully transferred into separating funnels and left for 24 h. This step was conducted to ensure the effective separation of glycerol from the generated FAME (Fatty acid methyl ester) as a dense layer at the bottom of the funnel. The FAME formed after the isolation of the glycerol layer was heated for 5 h at 70 °C to evaporate the unreacted free methanol molecules. The concentration of the generated FAME was determined using the gas-chromatography technique (Agilent 7890A). The values of FAME detected were directly used to calculate the biodiesel yields based on equation (1). The analysis of the samples was accomplished after their dilution with n-hexane and accompanied by methyl heptadecanoate as an internal standard. The gas-chromatograph device used has an injector (split/splitless), detector (flame ionization), and capillary column ((DB WAX) 30 m × 0.25 m × 0.25 μm) of H2 carrier.

The second-order polynomial equation (equation (2)) was used to represent the influence of the parameters in the biodiesel yields and the target responses.

where Y is the biodiesel response, Xi and Xj are the input parameters, β0 is a constant, βi is the coefficient linear term, βii is the coefficient quadratic term, βij is the coefficient cross-product term, and K is the number of parameters.
3. Results and discussion
3.1. Characterization of the Na+/clino catalyst
3.1.1. XRD analysis
The natural zeolite sample exhibits a pattern of several recognizable peaks at about 9.88°, 11.18°, 13°, 14.9°, 17.36°, 17.5°, 19°, 22.36°, 22.7°, 25°, 26°, 26.87°, 28.1°, 30°, 32°, 32.9°, and 34° which characterize the clinoptilolite species (figure 1(A)). This reflects the existence of clinoptilolite as a single phase in the natural zeolite sample with a 56 nm average crystallite size. For the green Na+/Clino, the pattern displays strong changes in the identified crystalline phases and an obvious impact on the crystalline structure of clinoptilolite (figure 1(B)). The crystalline phase of clinoptilolite is confirmed by its peaks, which are shifted to lower positions (22.35°, 25.56°, and 29.97°); this a significant indicator of distortion in the crystal lattice of the clinoptilolite or a partial alteration in its structure (figure 1(B)). Additionally, new peaks are observed at 19.1°, 31.84°, and 33.85° which are identified as the distinguishing peaks of sodium silicate (JCPDS No. 16-0815) (figure 1(B)). The formation of the sodium silicate phase is essentially related to the green alkaline alteration of the aluminum silicate structure of clinoptilolite. This gives the structure enhanced value, as it has higher basicity than the raw clinoptilolite.
Figure 1. The XRD patterns of raw clinoptilolite (A) and the green synthetic Na+/clinoptilolite (Na+/Clino) (B).
Download figure:
Standard image High-resolution image3.1.2. SEM and EDX analyses
The changes observed in the structure of clinoptilolite and the formation of new crystalline phases were associated with significant changes in the surficial morphologies. The clinoptilolite particles appeared as clusters of flaky compacted grains which commonly characterize this type of mineral (figures 2(A) and (B)). In the synthetic Na+/Clino, the original morphology of clinoptilolite was completely changed, and the particles appeared in well-developed bunches of several nano-clusters of globular form (figures 2(C) and (D)). Such attractive morphologies reflected the value of the green process in modifying the surficial features. Additionally, this is greatly effective in increasing the surface area of the modified product. After the modification process, the catalyst exhibits a particle size distribution from 40 nm to 120 nm with an average particle size of 64 nm.
Figure 2. SEM images and EDX analysis of raw clinoptilolite (A and B) and SEM images and EDX analysis of the green synthetic Na+/clinoptilolite (Na+/Clino) (C and D).
Download figure:
Standard image High-resolution imageThe EDX elemental analysis of raw clinoptilolite showed elemental contents of Si (36.28%), O (46.64%), K (3.43%), Al (7.21%), Fe (3.01%), and Ca (1.98%) (figure 2(B)). Following the green incorporation of sodium ions, the sodium content clearly increased to about 27.23%, which has a strong impact in increasing the basicity of the green product (figure 2(C)). Additionally, the Si and Al content decreased markedly, to nearly 8.48% and 1.5%, respectively. This demonstrates the strong substitution of such functional elements by sodium ions and suggests the possible leaching of Si ions and their incorporation into sodium silicate (figure 2(C)).
3.1.3. FTIR analysis
As a natural zeolite mineral, the FTIR spectrum of clinoptilolite reflected the presence of its essential functional groups of T–O and/or O–T–O (T = Al and Si) at 467 1/cm, the T–O bending of TO4 at about 1041 1/cm, zeolitic water at 1640 1/cm, and OH groups at 3442 1/cm [33, 34] (figure 3(A)). The spectrum of Na+/Clino also verified the existence of the same clinoptilolite chemical groups but at clearly shifted positions (figure 3(B)). This confirmed the presence of effective distortion in the structural units of clinoptilolite and changes in the mineral phases present. Moreover, the distinguishing band of OH was strongly intensified following the green entrapment of Na+ ions within the structure of clinoptilolite (figure 3(B)). Such an increment in the dominance of the OH groups was related to the etching effect of the alkaline solution on the aluminosilicate structure of zeolite, which induces exposure of the OH-bearing functional groups (siloxane) [35]. Therefore, the modification method used has a strong impact in enhancing the total basicity, which is a critical parameter for controlling the catalytic activity of clinoptilolite and the associated transesterification reaction.
Figure 3. The FTIR spectra of raw clinoptilolite (A) and the green synthetic Na+/clinoptilolite (Na+/Clino) (B).
Download figure:
Standard image High-resolution image3.1.4. Textural and physicochemical properties
The surface area, porosity, ion exchange capacity, and the total basicity were determined to be the controlling textural and physicochemical factors affecting the efficiency of heterogeneous catalysts (table 1). However, the surface area was enlarged, from 258 m2 g−1 for clinoptilolite, to 312 m2 g−1 for Na+/Clino; both the pore diameter and the pore volume showed a noticeable decrease in their values with the green doping of Na+ ions (table 1). The reported morphology of Na+/Clino is an essential factor that increased its surface area, compared to clinoptilolite as a raw mineral. The low pore diameter and the pore volume of Na+/Clino, considering the determined values for clinoptilolite, might be related to the effect of the newly formed sodium silicate phase in filling the structural channels of zeolite.
Table 1. The textural and physicochemical parameters of clinoptilolite and Na+/Clino.
Parameters | Clinoptilolite | Na+/Clino |
---|---|---|
Specific Surface area (m2 g−1) | 258 | 312.7 |
Total volume (ml g−1) | 0.041 | 0.038 |
Average pore size (nm) | 18.3 | 17.3 |
Cation exchange capacity (meq/100 g) | 132 | 387 |
Basicity (mmol OH/g) | 3.86 | 6.41 |
The values of the ion exchange capacity and the total basicity of Na+/Clino are significantly higher than those determined for mineral clinoptilolite (table 1). The effect of the modification reactions in distorting the structure of clinoptilolite, the associated leaching of Si and Al ions and/or their substitution by Na+ ions, and the formation of a sodium silicate phase improved the ion exchange capacity. Also, the entrapped Na+ ions, in addition to the etching effect of the sodium-bearing solution on the siloxane groups of clinoptilolite, primarily contributed to the basicity of the final product (Na+/Clino).
3.2. Response surface studies of biodiesel production
3.2.1. Analyses of the variances and validation of the approach
Given a regression analysis of the variance (ANOVA) function of the design used, the validation of the second-order quadratic polynomial model as a descriptive model of the biodiesel production tests can be confirmed. The design involved a series of transesterification experiments using randomly selected values of the significant factors (A. experimental interval, B. Na+/Clino loading, C. temperature, and D. methanol-to-palm-oil ratio) (table 2).
Table 2. The experimental runs of the design and the determined value of the response (biodiesel yield).
Biodiesel yield (%) (Y) | Ethanol to oil ratio (%) (D) | Temperature (°C) (C) | Na+/Cino loading (wt%) (B) | Time (min) (A) | Run | Std |
---|---|---|---|---|---|---|
11 | 1 | 40.00 | 4.00 | 50.00 | 15.00 | 60.8 |
5 | 2 | 40.00 | 1.50 | 100.00 | 10.00 | 62.3 |
21 | 3 | 70.00 | 2.75 | 50.00 | 12.50 | 84.8 |
4 | 4 | 100.00 | 4.00 | 50.00 | 10.00 | 58.7 |
19 | 5 | 70.00 | 1.50 | 50.00 | 12.50 | 77.6 |
7 | 6 | 40.00 | 4.00 | 100.00 | 10.00 | 67.9 |
22 | 7 | 70.00 | 2.75 | 100.00 | 12.50 | 96.4 |
16 | 8 | 100.00 | 4.00 | 100.00 | 15.00 | 70.3 |
23 | 9 | 70.00 | 2.75 | 75.00 | 10.00 | 85.7 |
1 | 10 | 40.00 | 1.50 | 50.00 | 10.00 | 58 |
2 | 11 | 100.00 | 1.50 | 50.00 | 10.00 | 53.2 |
15 | 12 | 40.00 | 4.00 | 100.00 | 15.00 | 74.6 |
25 | 13 | 70.00 | 2.75 | 75.00 | 12.50 | 90.3 |
20 | 14 | 70.00 | 4.00 | 75.00 | 12.50 | 88.6 |
18 | 15 | 100.00 | 2.75 | 75.00 | 12.50 | 84.6 |
24 | 16 | 70.00 | 2.75 | 75.00 | 15.00 | 82.2 |
14 | 17 | 100.00 | 1.50 | 100.00 | 15.00 | 68.6 |
12 | 18 | 100.00 | 4.00 | 50.00 | 15.00 | 58.2 |
13 | 19 | 40.00 | 1.50 | 100.00 | 15.00 | 71.3 |
9 | 20 | 40.00 | 1.50 | 50.00 | 15.00 | 65.8 |
3 | 21 | 40.00 | 4.00 | 50.00 | 10.00 | 63.4 |
6 | 22 | 100.00 | 1.50 | 100.00 | 10.00 | 60.3 |
26 | 23 | 70.00 | 2.75 | 75.00 | 12.50 | 90.3 |
8 | 24 | 100.00 | 4.00 | 100.00 | 10.00 | 65.3 |
17 | 25 | 40.00 | 2.75 | 75.00 | 12.50 | 80.3 |
10 | 26 | 100.00 | 1.50 | 50.00 | 15.00 | 62.6 |
Based on the resulting fitting degree (determination coefficient >0.97) for the biodiesel yields obtained from the actual experiments and the theoretical values, the selected polynomial model is of excellent significance in representing the tests conducted and the associated behaviors (figure S1(A)). This was supported by addressing the regression relation of the studentized residuals that also declared normal properties in predicting and representing the responses (biodiesel yield) (figure S1(B)). Additionally, the prediction deviation curve for all the experiments confirmed the excellent predictive accuracy of the selected polynomial model (figure 4). It was observed that the deviation of the determined responses exhibits a regular distribution around the reference line, either on the negative side or the positive side, within the range from −3.46 to 4.44 (figure 4).
Figure 4. The standard deviation values of the biodiesel yields obtained for the 26 tests undertaken.
Download figure:
Standard image High-resolution imageThe significance and the validation of the model were also investigated based on the essential ANOVA parameters (model-F, the sum of squares, lack of fit, and model-Prob >F) (table S2). The model-F value (29.06) is a strong indicator of the significance of the model and displays a noise effect of no more than 0.01% (table S2). The confirmation by the model-Prob-F of values lower than 0.05 for all the factors emphasizes the significance of these factors and the nonlinear relation between them and the studied response (biodiesel yield) (table S2). Additionally, the observed agreement between Pred R-Squared (0.87), Adj R-Squared (0.91), and Adeq Precision (16.82) confirms the presence of an adequate signal and the suitability of the quadratic polynomial model for navigating the design space (table S2).
Based on the previously reported significance of the model, the relation between the operating factors (A. experimental interval, B. Na+/Clino loading, C. temperature, and D. methanol-to-palm-oil ratio) and the response (Y. biodiesel yield) can be described by the following polynomial regression (equation (3)).
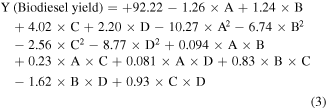
3.2.2. Influence of the significant variables and their interactions
3.2.2.1. The time interval and temperature
The impact of the interaction between the suggested intervals and temperature values on the heterogeneous transformation of palm oil into FAME (biodiesel) over Na+/Clino was emphasized by the 3D regression curve (figure 5(A)). The impact was assessed by setting the other essential factors to their intermediate values (2.75 wt% Na+/Clino loading and a 12.5:1 methanol-to-palm-oil molar ratio).
Figure 5. The effects of interactions between the essential transesterification variables on the biodiesel yield; (A) the test time interval and the adjusted temperature, (B) the test time interval and Na+/Clino loading, (C) the test time interval and the methanol-to-oil ratio used, (D) the adjusted temperature and the Na+/Clino loading, (E) the adjusted temperature and methanol-to-oil ratio, and (F) the methanol-to-oil ratio used and the Na+/Clino loading.
Download figure:
Standard image High-resolution imageThe data presented show augmentation of the detected biodiesel yields with an increase of the experimental period from 40 min to 100 min at all the suggested temperature values (50 °C, 75 °C, and 100 °C) (figure 5(A)). Considering the temperature at its lowest level (50 °C), the yield was noticeably upgraded from 76.7% to 84.8% by extending the period from 40 min to 70 min. At 75 °C, the observed yield shows an upgrade from 80.3% to 90.3% when the reaction interval is extended from 40 min to 70 min. The highest temperature (100 °C) increased the yield obtained from 84.4% to 96.4% when the transesterification interval was extended from 40 min to 70 min. Therefore, the temperature has a positive influence by intensifying the catalytic performance of Na+/Clino as a function of the elapsed time interval, especially at 100 °C.
The previously reported impact of temperature was credited to the properties of the transesterification process; as an endothermic chemical reaction, high-temperature conditions are ideal for accelerating the conversion rate [18]. Additionally, the temperature reduces the impact of the oil's viscosity as well as the immiscibility between the palm oil and the utilized methanol, which supports their homogeneity and increases the probability of interaction [2]. Moreover, the homogeneity and miscibility properties can be significantly improved by increasing the reaction time period [36].
In the Na+/Clino transesterification system for palm oil, experiments that took more than 70 min showed adverse effects for such time intervals. After 100 min, the experiments resulted in yields of 73.8%, 84.6%, and 82.3% given temperatures of 50 °C, 75 °C, and 100 °C, respectively (figure 5(A)). This behavior has been widely reported in the literature, and is result of the reversible nature of the transesterification processes. An over-expansion of the experimental period commonly causes hydrolysis of the fatty-acid esters generated, regenerating the original free fatty acids [18, 37]. Based on the reported interaction effects, the best temperature and optimum time interval for the transesterification of palm oil over Na+/Clino are 100 °C and 70 min, respectively.
3.2.2.2. The time interval and Na+/Clino loading
The effect of the interaction of Na+/Clino loading with different time intervals was evaluated by setting the other factors to their intermediate levels (75 °C temperature and 12.5:1 methanol-to-palm-oil ratio). Based on the 3D curve, a regular increase of the Na+/Clino content from 1.5 wt% to 2.75 wt% has a significant influence in accelerating the reaction rate as a function of the time interval consumed (figure 5(B)). Additionally, expanding the interval has a strong influence in increasing the catalytic effect as a function of the Na+/Clino dosage, considering the reported best interval (figure 5(B)).
After 40 min, the recognized yields using 1.5 wt%, 2.75 wt%, and 4 wt% of Na+/Clino are 75.13%, 80.3%, and 77.8 5, respectively. After 70 min, the determined yields for the same loading values, in order, are 84.2%, 90.3%, and 88.6%. After 100 min, the Na+/Clino catalyst achieved yields of 72.8%, 84.6%, and 75.11% when its loading was increased by 1.5 wt%, 2.75 wt%, and 4 wt%, respectively. Based on these data, the positive interaction effect of Na+/Clino loading extends from 1.5 wt% to 2.75 wt% and its use at a loading of 4 wt% has a slight adverse effect. Generally, higher Na+/Clino loading is associated with an increase in the exposed surface area of its particles as well as the active catalytic sites, which strongly accelerates the reaction, resulting in promising yields [3, 38]. However, this is essentially controlled by the available space and the volumes of the liquid phases used. Therefore, the saturation of the liquid phase with an excessive content of Na+/Clino particles has the direct effect of increasing the reactants' viscosity and the mass transfer resistance, which negatively affect the efficiency of such heterogeneous catalytic reactions [1, 18].
3.2.2.3. The time interval and methanol-to-palm-oil ratio
The 3D curve for the influence of the methanol-to-palm-oil ratio on the interaction at different suggested intervals was obtained by setting the temperature and Na+/Clino loading at their intermediate values of 75 °C and 2.75 wt%, respectively (figure 5(C)). Conducting the experiments for 40 min resulted in biodiesel yields of 72.3%, 80.3%, and 76.5% in the presence of methanol at molar ratios of 10:1 (methanol:palm oil), 12.5:1 (methanol:palm oil), and 15:1 (methanol:palm oil), respectively. After 70 min, the observed yields were enhanced to 85.7%, 90.3%, and 82.2% for the previously reported ratios, respectively. After 100 min, the values declined to 69.6%, 84.6%, and 74.2% using the methanol ratios of 10:1, 12.5:1, and 15:1, respectively.
Based on the results, raising the alcohol content to 12.5:1 as the molar ratio of alcohol to palm oil has a strong positive effect on the efficiency of the reaction as a function of the time, using the previously determined best time interval (70 min). The methanol molecules are essential radicals in the formation of FAME (biodiesel) during the chemical reaction with the free fatty acids of the used oil, which explains the experimental improvement in the yields associated with an upgrade of the methanol content [38]. Additionally, adjusting the molar ratio has a strong role in directing the balance of the reaction and reducing both the immiscibility and the mass transfer resistance [19].
Besides the positive influence of the methanol content at its optimal ratio, an excess of it has an adverse influence, as experimentally detected at the studied ratio of 15:1. Several reasons are suggested for this behavior, including the following: (1) an excess of free alcohol can cause deactivation of the surface of Na+/Clino as a catalyst; (2) such an excess of methanol interrupts the reaction and its balance; (3) the presence of methanol as unreacted or free molecules dissolves the glycerol molecules, causing a reversible effect, and (4) the predicted inversion in the polar groups of such alcohol molecules has an emulsifying impact [13, 14, 16].
3.2.2.4. Temperature and Na+/Clino loading
The influence of the interaction of Na+/Clino loading in inducing the conversion of palm oil as a function of the suggested temperature values was measured using a reaction time of 40 min and 12.5:1 as the molar/palm oil ratio (figure 5(D)). At 50 °C, the yields of FAME obtained from palm oil over Na+/Clino at loadings of 1.5 wt%, 2.75 wt%, and 4 wt% were 77.6%, 84.8%, and 79.3%, respectively. At 75 °C, the yields obtained for the same Na+/Clino loadings were 84.2%, 90.3%, and 88.6%, respectively. At 100 °C, the values were significantly upgraded to 84.9%, 96.4%, and 89% for the previously mentioned Na+/Clino loadings, respectively. These results emphasize the strong effects of temperature in promoting the catalytic efficiency of Na+/Clino as a function of its loading. Additionally, the results demonstrated that the best impact due to temperature can be achieved for a loading of 2.75 wt% of Na+/Clino.
3.2.2.5. The temperature and methanol-to-oil ratio
The impact of the interaction of temperature at the suggested molar ratios of methanol to palm oil was assessed using the intermediate values of the experimental time interval (70 min) and Na+/Clino loading (2.75 wt%) (figure 5(E)). At 50 °C, the FAME yields resulting from palm oil over Na+/Clino at methanol-to-oil molar ratios of 10:1, 12.5:1, and 15:1 were 75.6%, 84.8%, and 78.14%, respectively. At 75 °C, the yields determined for the same molar ratios were 85.7%, 90.3%, and 82.2%, respectively. At 100 °C, the values were significantly upgraded to 81.7%, 96.4%, and 88% for the previously mentioned molar ratios, respectively. This revealed a substantial intensification of the catalytic effect of the methanol content with increasing temperatures. Also, the maximum impact of temperature can be seen at a 12.5:1 molar ratio of methanol to palm oil.
3.2.2.6. Methanol-to-oil ratio and Na+/Clino loading
The impact of the interaction of methanol ratios at different Na+/Clino loadings was measured using the intermediate values of the experimental interval (70 min) and temperature (75 °C) (figure 5(F)). Using methanol at a 10:1 molar ratio, the resulting FAME yields were 71.6%, 85.7%, and 77.4% for Na+/Clino loadings of 1.5 wt%, 2.75 wt%, and 4 wt%, respectively. For the 12.5:1 molar ratio, the determined yields for the same Na+/Clino loadings were 84.2%, 90.3%, and 88.6%, respectively. For the 15:1 molar ratio, the determined values were 79.3%, 82.2%, and 78.5% for the previously mentioned Na+/Clino loadings, respectively. The observed yields revealed a favorable role for the methanol content in improving the activity of Na+/Clino as a function of its loading value within a range from 10:1 to 12.5:1 as molar ratio.
3.2.3. Statistical optimization
Based on the predicted quadratic programming options and the optimization function of the Design-Expert software (Version 6.0.5), the solutions predicted to optimize the transesterification of palm oil over Na+/Clino are presented in table 3. The suggested optimal solutions were controlled by the minimum and maximum limits for the significant factors, in addition to the scheme constraints, which were adjusted for the optimization tests (table 3). The suggested solutions reflected strong agreement between the best experimental conditions and the conditions predicted statistically by the design (table 3). Additionally, the suggested conditions can enhance the obtained yield to 98.2% when adjusted to a test interval of 85 min, a Na+/Clino loading of 3 wt%, a 12:1 methanol-to-oil ratio, and a temperature of 100 °C (table 3).
Table 3. The scheme constraints of the optimization tests and suggested optimal solutions for the best biodiesel yields from palm oil over the Na+/Clino catalyst.
Optimization test scheme constraints | ||||||
---|---|---|---|---|---|---|
Name | Lower limit | Upper limit | Lower coded | Upper coded | Importance | |
Time | Is in range | 40 min | 100 min | 1 | 1 | 3 |
Temperature | Is in range | 50 °C | 100 °C | 1 | 1 | 3 |
Methanol:oil ratio | Is in range | 10:1 | 15:1 | 1 | 1 | 3 |
Catalyst loading | Is in range | 1.5 wt% | 4 wt% | 1 | 1 | 3 |
Suggested optimization tests | ||||||
Solution number | Time (min) | Loading (wt%) | Temperature (°C) | Methanol:oil ratio (%) | Biodiesel yield (%) | Desirability |
1 | 70 | 2.75 | 100 | 12.5:1 | 96.4 | 1.000 |
2 | 85 | 3 | 100 | 12:1 | 98.2 | 1.000 |
3 | 75 | 2.7 | 90 | 12.5:1 | 95.7 | 1.000 |
4 | 75 | 3 | 100 | 12:1 | 97.3 | 1.000 |
3.3. Recyclability
The recyclability of heterogeneous catalysts is a critical factor for their further assessment for commercial production. The Na+/Clino particles used were separated and carefully washed three times with methanol as the recommended high-efficiency organic solvent for dissolving the oil and glycerol residues on the surface of the catalyst. The washing process consumed about 15 min each time and the washed product was then dried at 80 °C for 12 h to be ready for further cycles of use. The six recyclability tests were completed at fixed conditions of 70 min, 2.75 wt%, 12.5:1, and 100 °C for the test period, the Na+/Clino loading, the methanol-to-palm-oil ratio, and the temperature, respectively (figure S2).
Based on the measured yields, as a catalyst, Na+/Clino has excellent recyclability properties during the palm-oil-to-FAME transesterification reaction (figure S2). It achieved a biodiesel yield higher than 95% in the first two recyclability cycles, more than 92.5% for four recyclability cycles, and more than 90% for six recyclability cycles (figure S2). The decrease in the experimentally achieved yields following repeated recyclability tests is evidence of the effects of palm oil or glycerol over-adsorbed by the Na+/Clino active sites, hindering the catalytic effect [3].
3.4. Technical and safety properties
The technical specifications of the biodiesel sample obtained from palm oil using the Na+/Clino catalyst were appraised using the international requirements of the ASTM D-6751 and EN 14214 standards (table 4). Technically, the sample obtained strongly matches the recommended specifications of the biodiesel standards, including those for viscosity and density (table 4). The measured cetane index, as an important safety factor for biodiesel, had a value higher than 45, which qualifies this sample to be used as a safe and suitable fuel in engines with no, or few, hazardous emissions [3]. Moreover, the measured flash point is within the range of the safe fuels that can be handled and transported safely (table 4).
Table 4. The technical qualification of the biodiesel sample obtained from palm oil over a Na+/Clino catalyst.
Contents | Unit | ASTM D-6751 | EN 14214 | Biodiesel |
---|---|---|---|---|
Viscosity | mm2 s−1 | 1.9–6 | 3.5–5 | 4.32 |
Moisture content | Wt(%) | <0.05 | <0.05 | 0.041 |
Flash point | °C | >93 | >120 | 171.3 |
Calorific value | MJ kg−1 | -------- | > 32.9 | 50.8 |
Cloud point | °C | −3 to 15 | -------- | 7.12 |
Pour point | pp | −5 to 10 | -------- | 6.22 |
Cetane number | ---------- | ≥47 | ≥51 | 54.6 |
Density | g cm−3 | 0.82–0.9 | 0.86–0.9 | 0.88 |
Na + K | mg Kg−1 | ≤5 | ≤5 | 3.52 |
Acid value | Mg/KOH/g | ≤0.5 | ≤0.5 | 0.42 |
Iodine value | ---------- | --------- | ≤120 | 90.5 |
Regarding the safety value of the catalyst used, the catalyst is based on clinoptilolite, a natural zeolite mineral with high chemical and mechanical stability, and low leaching properties. The modification technique used involved a green chemical process which mainly results in safe particles coated with biophenols and with biodegradable properties. The catalyst has significant reusability that reduces the amount of solid byproducts or solid wastes produced. Additionally, following deactivation of the spent catalyst, it is a promising product saturated with different active adsorption sites, which qualifies it be reused in refining wastewater or to be used as a barrier layer in waste-disposal sites.
3.5. Mechanism
The catalyst studied is a modified form of clinoptilolite with a Na+ doped chemical structure and a secondary phase of sodium silicate formed as an alteration product (figure 6). The modified clinoptilolite and the sodium silicate phase have a Na+-rich chemical structure, providing them with several basic sites for effective transesterification reactions. The green Na+ doping of clinoptilolite increased its reactivity and basicity, either by the leaching of its functional Si and Al ions, or by the etching of its siloxane groups. The K+ and Na+ ions in the structure of modified clinoptilolite have a controlling effect on the mechanism of the reaction. During the reaction, the methanol molecules were first adsorbed by the external receptor sites of Na+/Clino and this process also involves also strong abstraction of the hydrogen protons of the adsorbed methanol molecules (figure 6). Then, an exchange process occurred between the alkali ions (Na+ and K+) and the methanol molecules, producing CH3O– (an alkoxide anion). At the same time, a portion of the palm oil free fatty acids were adsorbed by the Na+/Clino, forming types of physical bond between the siloxane groups (Si–OH and Al–OH) of the clinoptilolite and the carbonyl groups of the oil. Subsequently, the alkoxide anions (CH3O–) attacked the carbonyl groups following the protonation effect, forming FAME (biodiesel) in the system [18] (figure 6). Additionally, the acidity of the methanol molecules which were adsorbed by Na+/Clino induced the formation of methoxide moieties (Step1) (figure 6).
Figure 6. The suggested transesterification mechanism of castor oil using the green synthetic Na+/clinoptilolite (Na+/Clino) as basic catalyst.
Download figure:
Standard image High-resolution image3.6. Comparison with other catalysts
The efficiency of the produced Na+/Clino green catalyst in the transesterification of palm oil was compared with other catalysts of heterogeneous and homogenous phases (table 5). The results emphasized in the comparison demonstrated higher activity for Na+/Clino than for several heterogeneous catalysts, such as BaO, SrO, CaO, KOH/bentonite, CaO/activated carbon, SO4-ZrO2, and Sr/ZrO2. Additionally, the catalyst has higher efficiency than some homogenous catalysts, such as KOH, NaOH, and Et3NHSO4. This emphasized the value of the green Na+ doping process in producing an innovative basic catalyst with environmental properties and a higher catalytic performance than several synthetic catalysts. This qualifies it to be effectively applied in the transesterification of different species of edible and nonedible oils.
Table 5. Comparison between the catalytic activity of the Na+/Clino catalyst and other catalysts described in the literature as a function of the obtained yields.
Heterogeneous catalysts | ||||||
---|---|---|---|---|---|---|
Catalyst | Time | T (°C) | Methanol-to-oil ratio | Catalyst loading (wt%) | Yield (%) | References |
Coconut coir husk derived catalyst | 180 min | 130 | 12:1 | 10 | 89.8 | [39] |
Ostrich eggshell- CaO | 60 min | 60 | 9:1 | 8 | 92.7 | [40] |
Cesium/ silica | 180 min | 65 | 20:1 | 3 | 90 | [41] |
BrO / SrO | 50 min | 65 | 9:1 | 2.8 | 95 | [42] |
Sr/ZrO2 | 87 min | 115 | 29:1 | 2.7 | 79.7 | [43] |
CaO–CeO2 | 240 min | 65 | 12:1 | 5 | 95 | [44] |
Rice husk ash-based catalysts | 240 min | 65 | 9:1 | 7 | 91.5 | [45] |
SO4-ZrO2 | 10 min | 250 | 25:1 | 0.5 | 90 | [46] |
BaO | 60 min | 65 | 15:1 | 3 | 95 | [47] |
Activated carbon/CaO | 80 min | 190 | 15:1 | 5.5 | 80.9 | [43] |
KOH bentonite | 180 min | 60 | 6:1 | 3 | 90.7 | [48] |
CaO | 60 min | 65 | 15:1 | 3 | 77.3 | [47] |
SrO | 60 min | 65 | 15:1 | 3 | 95 | [47] |
NaOH | 30 min | 60 | 6:1 | 1 | 97 | [49] |
Et3NHSO4 | 180 min | 170 | 15:1 | 5.2 | 82.1 | [50] |
KOH | 15 min | 60 | 9:1 | 1 | 93 | [51] |
KOH | 15 min | 60 | 9:1 | 1 | 89 | [51] |
Na+/Clino (Experimental) | 70 min | 100 | 12.5:1 | 2.75 | 96.4 | This study |
Na+/Clino (Theoretical) | 85 min | 100 | 12:1 | 3 | 98.2 | This study |
4. Conclusions
A green basic catalyst for the potential transesterification of palm oil into biodiesel was produced by the green doping of clinoptilolite with Na+ ions (Na+/Clino) using green tea extract and sodium nitrite salt. The green doping reactions improved the basicity (6.41 mmol OH/g), ion exchange capacity (387 meq/100 g), and surface area (312.7 m2 g−1), which enhanced the qualification of Na+/Clino to be effectively applied in heterogeneous transesterification reactions. A statistical central composite design was used to improve its efficiency for the transesterification of palm oil and to optimize the biodiesel production conditions. Based on the assessed experimental conditions and the interactions between the significant factors, the Na+/Clino realized a biodiesel yield of 96.4% after 70 min at 100 °C in the presence of 2.75 wt% catalyst loading, at a 12.5:1 methanol-to-palm-oil ratio. Theoretically, the yield can be upgraded to 98.2% by expanding the test time to 85 min and the loading value to 3 wt%. This demonstrates the promising catalytic performance of clinoptilolite after the green doping of its structure with Na+. Moreover, the biodiesel generated using this catalyst meets the appropriate technical qualifications based on international recommendations.
Acknowledgments
The authors extend their appreciation to the Deputyship for Research & Innovation, Ministry of Education in Saudi Arabia for funding this research work through project number IFKSURG-1440-047.