Abstract
The familiar bell–jar demonstration, commonly used to show that sound cannot travel in a vacuum, is revisited in this study. It is suggested that by emphasizing the different media present in this set-up, sound propagation in near-vacuum conditions can be made possible. In this way, the concept of sound propagation in this set-up can be examined in a nuanced manner. In a study involving upper-secondary students, the set-up has also been found to be helpful in addressing a few alternative conceptions related to sound propagation.
Export citation and abstract BibTeX RIS
1. Introduction
When presenting the wave nature of sound to physics students, teachers typically include the bell–jar demonstration as a means to highlight the role of a medium in sound propagation. In that activity, a sound source, usually a bell, is made to ring while air is being pumped out of the jar. The earliest record of this demonstration can be traced to the work of Athanasius Kircher (1602–80) [1]. A related experiment was done by Otto von Guericke (1602–86), who built a pump that was used to take out air from a pair of copper hemispheres [2], which commonly feature in physics textbooks as Magdeburg hemispheres. Both Kircher and von Guericke observed that even after taking out most of the air from a sealed container with a bell inside, the ringing of the bell remained audible. On the basis of this observation, they concluded that air is not needed for the transmission of sound [1]. This observation was perhaps due to the low efficiency of the pump that they used and the leakage of air in the containers used. Inspired by von Guericke, Boyle (1627–91) made a more efficient, yet simpler, pump with the help of Robert Hooke (1635–1703) [2]. Boyle wanted to examine how sound propagates in a vacuum. Boyle's notion of a vacuum was based on the work of Evangelista Torricelli concerning air pressure. In Toricelli's experiment, he inverted a tube filled with mercury, which left a space (i.e., vacuum) in the topmost portion of it [2]. Using his newly designed and much improved pump, Boyle carefully conducted his version of the bell-in-a-jar experiment and observed that the sound of an alarm clock placed inside a glass chamber gradually faded away as air was being pumped out of the chamber [3]. He concluded that air is required in the propagation of sound—a view which is consistent with the Aristotelian view, which emphasises the role of a material medium in the transmission of sound.
Some scholars have mentioned a few problems related to the use of the classic bell–jar demonstration [4–7, 9, 11].
First, even sophisticated vacuum pumps leave some air inside the jar, which is enough to allow for transmission of sound. In the paper by Fleming [7], he highlighted that at room temperature this residual air exerts a pressure of about 10−2 cm Hg (about 13 Pa); under these conditions, the mean free path of the air particles is about 7 × 10−4 m. He noted that if the frequency of the sound emitted by the bell is about 300 Hz, the wavelength comes to about 1.1 m. He emphasized that since the wavelength of the sound is greater than this mean free path of the air particles, the sound can still be transmitted by the air inside the jar. Sprott [8], however, clarified that when the mean free path of the air particles inside the jar exceeds a few centimetres in value, transmission of sound would effectively stop. He based this deduction on the idea that in order to achieve the stated value of the mean free path of the air particles, the pressure inside the jar needs to be reduced to about 10 mTorr—which cannot be achieved by ordinary vacuum pumps.
Second, there are other factors that produce more dominant effects than the low density of air in reducing the audibility of sound inside a bell–jar. In particular, the difference in impedance between air and glass (which makes up the bell–jar) tends to play a major role in this demonstration and interacts with the effect of reduced air pressure [4, 7, 9, 11]. When air density becomes very low, which occurs when air pressure is reduced to about 0.1 mm Hg (the minimum attainable by typical mechanical air pumps), the sound produced by the vibrating source may not be audible to a listener, not because of the absence of air but because of the large impedance mismatch produced by the large differences in densities between the vibrating source and air, and then between air and glass [4, 7, 9, 11]. At these interfaces, reflection of sound occurs, and thus the sound is inaudible outside the jar [4, 7, 9, 11]. To remedy the problem, Dejun [5] suggested adding a microphone inside the bell–jar to avoid the effect of the air–glass impedance mismatch. However, this approach was criticized by other authors. Gavenda [4] noted that a new problem on impedance mismatch arises between air and the microphone. Brody [10] highlighted that it may be difficult to justify that it is the lack of air and not the features of the microphone and its response to near-vacuum conditions that caused the reduced intensity of sound. He also added that sound may also propagate through the microphone stand. Swartz [11] wrote that as the pressure is progressively reduced inside the bell–jar, the difference in impedance between the air and the glass surface of the bell jar increases—which, according to Gavenda [1], means more sound is reflected off the glass and little is transmitted to the outside.
Given the issues cited above, is it still advisable to present the 362-year-old bell–jar experiment in the teaching of wave propagation to upper secondary physics students who do not have a deep background in acoustic impedance and the theory of wave propagation? What would the benefits be?
The results of our current survey and an earlier study by Caleon and Subramaniam [12] indicate the prevalent notion among upper-secondary students (15–16 years old) that the presence of air or actual movement of air from source to listener is a necessary condition for efficient sound transmission. In our survey of students' (N = 97) conceptions on waves, we asked the students whether they are likely to hear the sound from the sound source that rests on the base of an evacuated bell–jar (see figure 1). About 60% of our respondents espoused the view that, even in the presence of other media (i.e., solid metal base), sound cannot propagate when there is very little air available. In the study of Caleon and Subramaniam [12], 74% of the respondents (243 upper-secondary students) also supported this view, with high confidence. These authors also reported that 47% of their sample upheld the view that if air is trapped along with its source in a room, an outside listener cannot hear the sound because the main medium, which is air, cannot get out. About 30% of the students in our survey also espoused this alternative view, with the reasoning that air movement from the source to the receiver will be blocked by some material (e.g., solid wall) which, in turn, blocks the sound transmission. The view that 'sound may be locked in closed spaces' was also reported by Eshach and Schwartz [13], in relation to eighth-grade students. In the context of the bell–jar set-up, Eshach and Swartz found that many of the students they interviewed believed that 'sound is carried by the air' and that 'in the absence of air, sound cannot be carried from clock [source] to ears [receiver]' (p 758). Using a different set-up, Linder and Erickson [14] reported that tertiary students also support the view that sound is being physically carried by air molecules from source to receiver. Their subjects also expressed the related alternative view that 'sound pushes air as it propagates from source to receiver'. These authors mentioned that tertiary students' microscopic conceptions of sound propagation are indicative of the notion of a 'travelling medium' (p 499). This resonates with Hults's [15] observation: 'While studying wave behaviour, my students found it hard to comprehend that it is not the medium that travels, but only the wave ...' (p 671).
Figure 1. Typical bell–jar demonstration: sound source is hung by a string inside the bell–jar.
Download figure:
Standard imageThe foregoing research reports appear to be surprising for some physics teachers. Some teachers may assume that students would readily adopt the scientifically acceptable view—that air (medium) does not move along with sound (wave)—after exposing students to standard lectures on wave propagation. However, evidence to the contrary has been reported by several researchers who explored the details of how students conceive of the mechanism of sound propagation in air at a microscopic level. Our personal experience in teaching physics students and our observation of two physics classes show that students seem to have little difficulty in dealing with the scientifically acceptable view that 'sound can travel through other media' in some familiar contexts. For example, they readily profess that sound can travel through solids when asked whether they could hear sound when someone is tapping on a table and they placed their ears on it to listen to the sound generated. When shown an old tin-can telephone, they readily respond that sound can travel through the strings connecting the tin cans. However, students' difficulties become evident when applying this idea in other contexts, such as when multiple media are involved.
The stated alternative conceptions relevant to the bell–jar demonstration can be traced to how the topic of sound propagation is commonly presented in textbooks, internet sites and class lectures—most of them cite the bell–jar experiment as illustrative of the concept that sound cannot propagate in a vacuum. Without much elaboration, this discussion leaves an impression among students that there is no chance at all that sound can propagate in near-vacuum conditions (i.e., very low air pressure), even in the presence of other media. It is, however, worth noting that some students are able to grasp, intuitively, the notion of the reflection of sound while looking at the bell–jar set-up. In the survey that we conducted, students responded negatively when asked whether a listener could hear the sound of a clock ringing inside a glass bell–jar. They, however, correctly reasoned that the sound could be inaudible because the sound is reflected inside the glass walls of the bell–jar. This finding suggests that students' intuitive knowledge may correlate well with scientific conceptions, and it would be advisable to use this for 'hooks' in discussing the effect of impedance mismatch in sound propagation.
We feel that the bell–jar demonstration can be used to highlight the importance of different types of media, other than air, in wave propagation; and to help students to grasp, albeit indirectly, the notion that, for sound to propagate, air need not move along with the sound. Building upon the ideas of Villa [6], we thought about showing two versions of the demonstration to the students. The first version resembles the set-up of the classic bell–jar demonstration: it prevents transmission of sound from the cell phone to the bell–jar by hanging the former with a string (see figure 1). The second version (figure 2), which allows transmission of sound to the outside, has the cell phone placed on the metallic base of the bell–jar. In each version, the cell phone was activated using another cell phone. We made a video of the demonstrations. We also embedded figures 1 and 2 in a refutational text. A refutational text explicitly presents students' alternative conceptions and the corresponding scientific conceptions [16]. We asked the students who completed our survey of students' conceptions on waves to read the refutational text before watching the video.
Figure 2. Modified bell–jar demonstration: sound source placed on base of the bell–jar.
Download figure:
Standard imageAfter reading the text and watching the video featuring the modified bell–jar demonstrations, we found that there was a substantial increase in the percentage of students (from 65 to 87%) who supported the scientific view that 'sound from a vibrating source can be heard outside an airtight room as long as other media are available to transmit the sound'. Similarly, the percentage of our sample who supported the scientifically correct view that 'sound can propagate even if very little amount of air is present, as long as other media are present' increased from 35 to 76% after reading the text and watching the video. The percentage of students who supported the view that the presence of air is necessary for sound transmission in this context dropped dramatically from 60 to 20%.
It is worth mentioning that proper planning and caution must be exercised in demonstrations so as not to seed any alternative conceptions. In the study made by some researchers on the effectiveness of demonstrations, it was found that traditional demonstrations may not effectively help students understand the underlying concepts and do not correct alternative conceptions that they may have [17]. In the bell–jar demonstration, teachers could start by mentioning the goal of the demo-activity. If it is to show that a medium is needed in sound propagation, then the teacher must clearly specify the presence of different media present in the set-up. It needs to be emphasized that even in near-vacuum conditions, with a little ingenuity, something can be done to transmit sound, that is, by placing the source of sound on the solid metal base of the bell–jar. It is also important to note that a true vacuum is not achievable, but even with a small amount of air left in the jar, sound propagation would be highly attenuated and thus the sound from a vibrating source would be essentially inaudible. By doing this, the concept of sound propagation is examined well, producing a better understanding of how the presence of different media affects sound propagation. For high-ability upper-secondary students and A-level students who take physics, ideas about impedance mismatch between air and glass under low air pressure conditions inside the bell–jar as well as issues about the mean free path of the air particles under these conditions can also be brought up during teaching, not only to promote richer discourse in the class but also to provide a nuanced understanding of the bell–jar demonstration.
Acknowledgment
We thank the Office of Education Research for a research grant (OER 40/08 RS) for this study.
Biographies
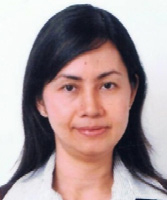
Imelda S Caleon is currently a research scientist at the Office of Education Research, National Institute of Education, Nanyang Technological University, Singapore. Her doctoral research focused on students' conceptualization of wave concepts. She holds a BSc in physics from De La Salle University, Philippines, and was a physics teacher for ten years.
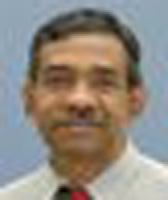
R Subramaniam is an associate professor at the National Institute of Education, Nanyang Technological University, Singapore. Physics education is among his diverse research interests.
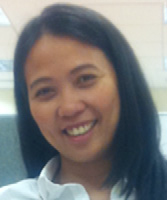
Ma Hershey P Regaya is a research assistant at the National Institute of Education, Nanyang Technological University, Singapore. She has an MSc in Physics from Ateneo de Manila University and taught secondary-level physics for more than ten years at the Philippine Science High School.