ABSTRACT
Large and heterogeneous isotopic variations of 150Sm/149Sm and 158Gd/157Gd due to neutron capture reactions caused by cosmic-ray irradiation were found in chemical and mineral separates from the Norton County meteorite. The light-colored separates, consisting mainly of enstatite (Mg2Si2O6), have a very large neutron fluence of 1.98 × 1017 n cm−2, which is 10 times higher than that of the whole rock. Furthermore, four chemical separates showed a large variation in neutron fluences, ranging from 1.82 × 1016 to 1.87 × 1017 n cm−2. The variable amounts of neutron fluences from a small single fragment of the Norton County meteorite cannot be simply explained by single-stage cosmic-ray irradiation in space. Rare earth element (REE) analyses revealed that the fractions with high neutron fluences have similar chemical properties to those in the early condensates in the solar system, showing depletions of Eu and Yb in their REE abundance patterns. The data provide evidence for an activity of the early Sun (T Tauri), suggesting the migration of early and intense irradiation materials into the Norton County meteorite's parent body.
Export citation and abstract BibTeX RIS
1. INTRODUCTION
Cosmic rays in space penetrate a few meters into the surface of planetary materials, and produce secondary nuclides, so-called cosmogenic nuclides, in the materials. The exposure history of meteorites has been characterized by several cosmogenic nuclides. Cosmogenic nuclides such as long-lived radioisotopes (10Be, 26Al, 36Cl, and so on) and stable noble gas isotopes (3He, 21Ne, 36Ar, 38Ar, and so on) have been widely used to determine the cosmic-ray exposure (CRE) ages of meteorites in space. Sm and Gd isotopic shifts by neutron capture reactions also provide useful information for understanding the exposure records of meteorites because 149Sm and 157Gd have very large thermal neutron capture cross sections and shift to 150Sm and 158Gd, respectively (Eugster et al. 1970; Hidaka et al. 1999, 2006).
Enstatite achondrites (aubrites) are highly reduced achondrite meteorites and must have formed in a very unique part of the solar nebula. Aubrites are coarse-grained rocks consisting mostly of nearly FeO-free enstatite, with minor and trace minerals very heterogeneously distributed throughout the rocks. Most aubrites generally show longer CRE ages than any other stony meteorites, and some of them are known to have experienced at least two exposures to cosmic rays, on their parent body and in space (Hidaka et al. 1999, 2006; Lorenzetti et al. 2003; Welten et al. 2004; Miura et al. 2007; Herzog et al. 2011). Furthermore, aubrites are known to have experienced numerous collisions, and most of them are brecciated. Some aubrites contain clasts that have a different origin to the matrices, such as ordinary-chondrite clasts in the Cumberland Falls host (Binns 1969). Regolith breccia-type aubrites such as Bustee, EET 90033, Khor Temiki, LEW 87007, Pesyanoe, and Y 793592 are known to contain solar-wind-implanted noble gases (Eberhardt et al. 1965; Marti 1969; Lorenzetti et al. 2003). In particular, Pesyanoe shows significant and heterogeneous isotopic variations of N, Ne, and Xe due to cosmic-ray irradiation, even in a small fragment (Müller & Zähringer 1966; Mathew & Marti 2003). Furthermore, Sm and Gd isotopic studies have revealed that the neutron fluences of four different phases taken from a small fragment of Pesyanoe show significant variation of up to 30% relative to the matrix portion (Hidaka et al. 2006). These results cannot be explained by single-stage cosmic-ray irradiation records of Pesyanoe, suggesting isotopic evidence for preirradiation effects in the record of the regolith before compaction into the meteorite parent body. Thus, the origin and the formation process of aubrites are still highly disputable.
Among the stony meteorites, the Norton County aubrite has one of the longest CRE ages: 111–115 Ma (Lorenzetti et al. 2003; Miura et al. 2007; Herzog et al. 2011). Considering that the recovery of the main mass is over 1000 kg, the size of the meteoroid is large enough to accumulate many kinds of cosmogenic nuclides. The large size allowed the secondary neutrons generated during the interactions to slow down to thermal energies before escaping into space. In addition, the low iron concentration also enhanced neutron capture effects because thermal neutrons stood a great chance of avoiding absorption by iron and of interacting with other elements. Therefore, Norton County is probably the best example to study neutron capture effects due to the interaction of cosmic rays with the planetary surface materials. There are some useful neutron-capture products such as 36Cl, 41Ca, 150Sm, and 158Gd from Norton County to enable consideration of the irradiation history of the Norton County meteorite. However, the production rates of individual neutron-capture products and the CRE ages are not always consistent. The discrepancy of neutron fluences estimated from neutron-capture produced 150Sm and 41Ca suggests the multi-stage irradiating history of Norton County (Welten et al. 2004; Herzog et al. 2011). Here we found very large isotopic shifts of Sm and Gd in specific phases of the Norton County meteorite, corresponding to 5–10 times higher neutron fluences than reported previously (Eugster et al. 1970; Hidaka et al. 1999, 2006; Herzog et al. 2011). In this paper, we will discuss the possible existence of preirradiation materials migrating into the meteorite body.
2. EXPERIMENTAL METHODS
2.1. Samples
The Norton County aubrite is a fragmental impact breccia consisting of coarse and angular enstatite crystal fragments, embedded into a clastic matrix (Okada et al. 1988). In this study, whole rock, handpicked material of the light-colored phase, and chemical separates obtained by a sequential acid-leaching method were prepared from the same single fragment of the Norton County meteorite weighing about 5 g.
Three whole rock samples (hereafter referred to as NCB1, NCB2, and NCB3) and the handpicked sample (NCW) weighing about 0.2 g were decomposed individually by HF–HClO4. The handpicked material of the light-colored phase was a concentrate of mainly enstatite crystals, as determined by electron probe micro-analysis.
Sequential acid-leaching treatments were carried out to obtain chemically different phases from the single samples. About 0.3 g of the powdered sample was leached using 5 mL of 0.1 M acetic-acid–ammonium acetate, 0.1 M HCl, 2 M HCl, and aqua regia, successively. Finally, the residue was decomposed by treatment with HF–HClO4 with heating. These five leaching fractions (NCL) are designated as 1, 2, 3, 4, and 5, respectively. The leaching experiments were performed twice. The fractions obtained from the first and second experiments were identified by using subordination numbers 1 and 2, respectively, after the 1–5 notation.
The samples, namely, three whole rocks (NCB1, NCB2, and NCB3), one light-colored phase (NCW), and 10 leachates (NCL#1–1 to NCL#5–2) were then taken to dryness and redissolved in 1 mL of 2 M HCl. The solutions were divided into two portions: the main portion for Sm and Gd isotopic measurements and the rest for the determination of REE abundances.
2.2. Mass Spectrometric Analyses
Most of the sample solution was used for the Sm and Gd isotopic measurements. Sm and Gd were separated by a conventional method using cation exchange methods (Hidaka & Yoneda 2007). The solution was loaded onto a cation exchange resin column and washed with 4.7 mL of 2 M HCl before the REE were eluted with 3 mL of 6 M HCl. The solution was then evaporated to dryness and redissolved in a drop of 0.1 M HCl. The solution was loaded onto a second column packed with LN resin to separate Sm and Gd using 0.35 and 0.5 M HCl, respectively. A Micro-mass VG54–30 thermal ionization mass spectrometer equipped with seven Faraday cup collectors was used for the isotopic measurements of Sm and Gd. Data collection was performed using the static multi-mode. However, seven collectors are not sufficient to simultaneously measure all seven isotopes of Sm and Gd and associated isobaric interferences. Therefore, in this study, 144Sm and 152Gd were not monitored for Sm and Gd isotopic analyses, respectively.
For Sm isotopic measurements, isobaric interference from Nd (144Nd, 148Nd, and 150Nd), and Gd (152Gd and 154Gd) are possible. The seven Faraday cup collectors were configured to monitor 146Nd, 147Sm, 148Sm, 149Sm, 150Sm, 152Sm, and 154Sm during the Sm measurements. In the measurement of background after every 10 data collection during the Sm analytical scheme, the contribution from Gd interference was checked at mass 155. However, no Gd interference was detected in all samples. Correction for the instrumental mass fractionation was performed using an exponential law normalized to 147Sm/152Sm = 0.56081 (Russ et al. 1972).
154Sm, 156Dy, 158Dy, and 160Dy can interfere with Gd isotopes. The collectors were configured to monitor 154Gd, 155Gd, 156Gd, 157Gd, 158Gd, 160Gd, and 161Dy. Contribution from Sm interference was checked at mass 150 during the background measurement. Since Sm usually evaporates at lower temperature than Gd by using thermal ionization mass spectrometry, no Sm interference was detected in all of the Gd measurements. For normalization of Gd isotopic ratios, 156Gd/160Gd = 0.9361 has been generally used (Eugster et al. 1970). However, the 156Gd/160Gd isotopic ratio cannot be fixed to a constant value for the neutron-irradiated materials because of the possible isotopic shifts from 155Gd to 156Gd. In order to solve this issue, modified 156Gd/160Gd ratio (0.9361+α) is used for the normalization factor, assuming that a depletion of 155Gd is equal to an enrichment of 156Gd (Hidaka et al. 1995; Hidaka & Yoneda 2007). From the isotopic data for non-irradiated material, the sum of 155Gd/160Gd and 156Gd/160Gd isotopic ratios is fixed to 1.61290. The α values in individual samples are determined when the normalization by 156Gd/160Gd = 0.9361+α leads to 155Gd/160Gd = 0.67680–α.
Another minor aliquot of each sample solution was once evaporated to dryness and redissolved using 5 mL of 0.5 M HNO3. A 0.05 g quantity of a 10 ppb indium solution was added to the individual sample solutions as an internal standard element to optimize the analytical conditions for REE measurements. ICP-MS (Agilent 7500cx) was used to determine the REE contents.
3. RESULTS AND DISCUSSION
3.1. Sm and Gd Isotopic Shifts
Among the samples of chemical separates, the isotopic data of fractions of NCL#3–NCL#5 from both two-times leaching experiments could not be obtained because of their low elemental abundances of Sm and Gd. All the other samples measured in this study show significant isotopic shifts of 150Sm/149Sm and 158Gd/157Gd. There is a significant difference in the Sm and Gd isotopic shifts, even in one of three whole rock samples, as shown in Table 1. The isotopic variation patterns of Sm and Gd illustrated in Figure 1 clearly show that the isotopic deviations come predominantly from neutron capture and are not from nucleosynthetic anomalies. Figure 2 shows correlation diagrams of isotopic shifts for (a) 149Sm/152Sm versus 150Sm/152Sm and (b) 157Gd/160Gd versus 158Gd/160Gd. These data give neutron fluences ranging from 1.89 × 1016 to 3.54 × 1016 n cm−2. In particular, one of three sets of whole rock data (3.54 × 1016 n cm−2) is 2.3–2.9 times higher than data reported previously (Hidaka et al. 1999, 2006), although the other two sets of data are almost similar to each other and to previous data. The variation in neutron fluences can be caused by different depths in the case of a large-sized meteorite body. Actually, in the case of Norton County, the neutron fluences of the specimens taken from different depths at 10–37 cm vary significantly; they range from 1.22 × 1016 to 3.1 × 1016 n cm−2 (Herzog et al. 2011). There is, however, no difference in depth among the samples used in this study. The three bulk samples were taken from a small fragment weighing about 5 g. Furthermore, the isotopic variations of Sm and Gd in the acid-leachates and the hand-picked sample are more heterogeneous and greater than those in the whole rock samples, corresponding to neutron fluences ranging from 1.82 × 1016 to 19.8 × 1016 n cm−2.
Figure 1. Isotopic variation patterns of Sm and Gd of all analyzed samples relative to the average values measured for the terrestrial standard materials. εiSm and εjGd are defined as follows: εiSm = {(iSm/152Sm)sample/(iSm/152Sm)STD −1} × 10000 (i = 147, 148, 149, 150, 154) εjGd = {(jGd/160Gd)sample/(jGd/160Gd)STD −1} × 10000 (j = 154, 155, 156, 157, 158).
Download figure:
Standard image High-resolution imageFigure 2. Correlation of isotopic shifts between (a) 149Sm/152Sm and 150Sm/152Sm, and between (b) 157Gd/160Gd and 158Gd/160Gd. A solid line in each figure shows an ideal neutron capture line with a slope of −1.
Download figure:
Standard image High-resolution imageTable 1. Isotopic Compositions of (a) Sm and (b) Gd of Chemical Separates, Mineral Separate, and Whole Rocks from the Norton County Meteorites
Sample | 148Sm/152Sm | 149Sm/152Sm | 150Sm/152Sm | 154Sm/152Sm |
---|---|---|---|---|
(a) Sm | ||||
Standard material | ||||
STD | 0.420461 ± 5 | 0.516868 ± 5 | 0.275996 ± 2 | 0.850854 ± 7 |
Chemical separates | ||||
NCL#1-1 | 0.420461 ± 71 | 0.516377 ± 74 | 0.276441 ± 59 | 0.850860 ± 99 |
NCL#2-1 | 0.420455 ± 60 | 0.516384 ± 81 | 0.276391 ± 67 | 0.850835 ± 97 |
NCL#1-2 | 0.420432 ± 10 | 0.515692 ± 11 | 0.277291 ± 12 | 0.850853 ± 21 |
NCL#2-2 | 0.420461 ± 78 | 0.512599 ± 58 | 0.280385 ± 76 | 0.850836 ± 87 |
Mineral separate | ||||
NCW | 0.420444 ± 81 | 0.512304 ± 71 | 0.280616 ± 84 | 0.850882 ± 81 |
Whole rocks | ||||
NCB1 | 0.420438 ± 28 | 0.516449 ± 22 | 0.276451 ± 18 | 0.850875 ± 34 |
NCB2 | 0.420435 ± 11 | 0.516109 ± 9 | 0.276873 ± 9 | 0.850842 ± 16 |
NCB3 | 0.420472 ± 77 | 0.516429 ± 79 | 0.276459 ± 80 | 0.850898 ± 15 |
References | ||||
Whole rock (Hidaka et al. 1999) | 0.420483 ± 4 | 0.516511 ± 3 | 0.276373 ± 3 | 0.850821 ± 7 |
Whole rock (Hidaka et al. 2006) | 0.420443 ± 9 | 0.516511 ± 10 | 0.276375 ± 7 | 0.850872 ± 17 |
Whole rock (Herzog et al. 2011) | 0.515881 ∼ 0.516417 | 0.276312 ∼ 0.276928 | ||
Sample | 154Gd/160Gd | 155Gd/160Gd | 157Gd/160Gd | 158Gd/160Gd |
(b) Gd | ||||
Standard material | ||||
STD | 0.099747 ± 2 | 0.676875 ± 5 | 0.715878 ± 4 | 1.135846 ± 7 |
Chemical separates | ||||
NCL#1-1 | 0.099789 ± 91 | 0.674440 ± 94 | 0.714743 ± 112 | 1.136928 ± 108 |
NCL#2-1 | 0.099770 ± 80 | 0.676466 ± 84 | 0.714842 ± 83 | 1.136876 ± 93 |
NCL#1-2 | 0.099772 ± 70 | 0.675283 ± 135 | 0.714280 ± 123 | 1.137429 ± 123 |
NCL#2-2 | 0.099790 ± 69 | 0.674783 ± 117 | 0.713191 ± 110 | 1.138542 ± 98 |
Mineral separate | ||||
NCW | 0.099795 ± 71 | 0.674476 ± 101 | 0.712800 ± 108 | 1.138898 ± 105 |
Whole rocks | ||||
NCB1 | 0.099780 ± 57 | 0.676449 ± 52 | 0.714775 ± 54 | 1.136937 ± 53 |
NCB2 | 0.099778 ± 61 | 0.676109 ± 69 | 0.714347 ± 42 | 1.137330 ± 58 |
NCB3 | 0.099766 ± 78 | 0.676430 ± 89 | 0.714738 ± 91 | 1.136994 ± 98 |
References | ||||
Whole rock (Hidaka et al. 1999) | 0.099761 ± 23 | 0.676463 ± 34 | 0.714818 ± 29 | 1.136870 ± 34 |
Whole rock (Hidaka et al. 2006) | 0.099768 ± 42 | 0.676494 ± 78 | 0.714843 ± 78 | 1.136889 ± 89 |
Whole rock (Eugster et al. 1970) | 0.09971 ± 4 | 0.67631 ± 30 | 0.71454 ± 19 | 1.13687 ± 25 |
Notes. Numbers as analytical errors of individual isotopic ratios are 2σ of the means of the last digits indicated.
Download table as: ASCIITypeset image
The chemical composition of the target material is yet another factor that alters the production rate of neutrons, and contributes to the variation in isotopic shifts of Sm and Gd. However, the neutrons produced in the target need to travel over long distances to reduce their energy to the thermal neutron level. Therefore, it is impossible to explain such an extremely large variation of the neutron production rate found in the three whole rock samples based on the difference of the chemical composition on the microscopic scale. The results are best explained by the migration of alien materials that had been highly irradiated into the Norton County matrix portion.
3.2. Neutron Thermalization
The calculation of cosmic-ray-produced neutron equilibrium spectra using the neutron transport equation was developed by Lingenfelter et al. (1972), hereafter denoted as the LCH model. The combination of Sm and Gd isotopic data from one sample is very useful for the determination of the neutron energy spectrum. The latter provides information on the sizes of meteoroids and the depths of meteorite samples during irradiation. In particular, the difference in neutron capture resonance between 149Sm (0.0973 eV) and 157Gd (0.0314 eV) can be effectively used to estimate the energy level of thermal neutrons (Lingenfelter et al. 1972). Since the resonance in 149Sm is at higher energy than that in 157Gd, the ratio of the two isotopic shifts of 150Sm/149Sm and 158Gd/157Gd is dependent on the neutron energy spectral shape and can be calculated as follows:
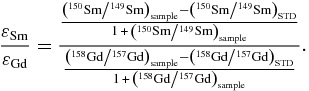
The value of εSm/εGd is therefore an indicator of the shape of neutron spectrum. The production rate of thermal neutrons also depends on the chemical composition and temperature of the target material (Lingenfelter et al. 1972). The chemical composition of the target is treated as the effective total macroscopic neutron capture cross section, Σeff (cm2 g−1), which is mainly estimated from the contents of Al, Ca, Fe, Mg, Si, and Ti as the major element component, and Sm, Eu, and Gd as the trace element component (Lingenfelter et al. 1972). In the LCH model, the value of εSm/εGd at different temperatures in the range from 0 to 400 K is predicted as a function of Σeff. The neutron energy spectrum proposed by the LCH model has been effectively used to discuss the exposure conditions of the planetary materials. Aubrites generally show higher neutron production rates than other meteorite species because they have lower contents of Fe and Ti as neutron absorbers of major element component in the rock sample. The macroscopic neutron capture cross section Σeff (cm2 g−1) is effectively used to characterize the chemical properties of the whole rock sample. Aubrites show lower Σeff values (0.0015–0.0023) than chondrites (0.0070–0.0096), lunar regolith (0.0042–0.0107), and so on (Hidaka et al. 1999). The estimated εSm/εGd, Σeff and the neutron fluence Ψ of individual fractions treated in this study are listed in Table 2.
Table 2. Summary of Parameters to Characterize Neutron Capture Reactions of the Norton County Samples
Sample | 150Sm/149Sm | 158Gd/157Gd | εSm/εGd | Ψ |
---|---|---|---|---|
(1016 n cm−2) | ||||
STD | 0.533970 ± 0.000006 | 1.586675 ± 0.000010 | 0 | |
NCL#1-1 | 0.535347 ± 0.000138 | 1.590681 ± 0.000291 | 0.58 ± 0.11 | 1.49 ± 0.03 |
NCL#2-1 | 0.535243 ± 0.000155 | 1.590389 ± 0.000226 | 0.57 ± 0.13 | 1.38 ± 0.03 |
NCL#1-2 | 0.537707 ± 0.000026 | 1.592413 ± 0.000324 | 1.09 ± 0.04 | 4.064 ± 0.005 |
NCL#2-2 | 0.546987 ± 0.000160 | 1.596405 ± 0.000282 | 2.24 ± 0.07 | 14.18 ± 0.03 |
NCW | 0.547753 ± 0.000181 | 1.597781 ± 0.000283 | 2.08 ± 0.06 | 15.01 ± 0.03 |
NCB1 | 0.535292 ± 0.000042 | 1.590513 ± 0.000141 | 0.56 ± 0.04 | 1.432 ± 0.008 |
NCB2 | 0.536462 ± 0.000020 | 1.592125 ± 0.000124 | 0.77 ± 0.02 | 2.708 ± 0.004 |
NCB3 | 0.535328 ± 0.000175 | 1.590784 ± 0.000245 | 0.55 ± 0.14 | 1.47 ± 0.03 |
References | ||||
Hidaka et al. (1999) | 0.535077 ± 0.000008 | 1.590433 ± 0.000080 | 0.49 ± 0.01 | 1.19 ± 0.02 |
Hidaka et al. (2006) | 0.535081 ± 0.000017 | 1.590404 ± 0.000214 | 0.51 ± 0.03 | 1.48 ± 0.04 |
Notes. Numbers as analytical errors of 150Sm/149Sm and 158Gd/157Gd are 2σ of the means. εSm/εGd: a parameter for neutron energy spectrum (see the text and Lingenfelter et al. 1972) given by the following equation:Ψ: neutron fluences estimated from 150Sm/149Sm isotopic ratios are given by the following equation:
where σSTD1, σSTD2, and σsample denote the effective neutron capture cross sections for the non-neutron-irradiated standard (Ψ = 0 n cm−2) for STD, for the neutron-irradiated standard of Ψ = 5.94 × 1015 n cm−2 for STD2 (Hidaka et al. 1999), and for each sample, respectively.
Download table as: ASCIITypeset image
A variation in εSm/εGd as a function of Σeff is interpreted to be the result of differences in the neutron energy spectra (Lingenfelter et al. 1972). In general, aubrites show εSm/εGd values ranging from 0.37 to 0.66 (Hidaka et al. 1999, 2006). A difference in Σeff values might produce a slight variation in εSm/εGd values. Figure 3 shows a variation in εSm/εGd as a function of Σeff, which is interpreted to be the result of differences in the neutron energy spectra. A shaded zone reveals calculated neutron energy spectra at a temperature in the range from 0 to 400 K based on the LCH model. For comparison, the data of representative extraterrestrial materials such as the Apollo lunar regolith (A-15, 16, and 17), aubrites and chondrites are cited (Hidaka et al. 1999, 2000a, 2000b, 2006; Hidaka & Yoneda 2007), and also plotted in the same figure. The samples with high neutron fluences, NCW and NCL#2-2, show much higher εSm/εGd values (2.08–2.24) than others (0.49–0.58). The data suggest that the two samples were irradiated by higher energy neutrons relative to thermalized neutrons. Interestingly, similar results are reported for the acid leachates of the Kapoeta meteorite (howardite), which is known as a solar-gas-rich meteorite (Hidaka & Yoneda 2009). High εSm/εGd values of the Kapoeta leachates (εSm/εGd = 1.9) indicate the possibility of the interaction with solar cosmic rays (SCR) and activity from the early Sun.
Figure 3. Diagram of effective macroscopic cross section (Σeff) vs. the balance of Sm and Gd isotopic shifts due to neutron capture (εSm/εGd) for various kinds of extraterrestrial samples. A shaded zone reveals calculated neutron energy spectra at a temperature in the range from 0 to 400 K based on the LCH model (Lingenfelter et al. 1972). The data points for closed symbols are in this study. The data points for open symbols are from references: aubrites (Hidaka et al. 1999, 2006); lunar regolith samples from the Apollo-15, 16, and 17 landing sites (Hidaka et al. 2000a; Hidaka & Yoneda 2007); chondrites (Hidaka et al. 2000b); and Kaopeta (Hidaka & Yoneda 2009).
Download figure:
Standard image High-resolution imageIn general, irradiation by galactic cosmic rays (GCR) produces secondary neutrons that are slowed down to thermal energy in a target material. Therefore, the neutrons produced by GCR require a certain distance to travel in the target and lose their energies down to the thermal level. The meteorites with large meteoroidal bodies such as Norton County are able to produce thermal neutrons by GCR irradiation. However, the coexistence of a variable energy type of neutrons in the small fragments, as shown in this study, is difficult to explain when based only on simple GCR irradiation results. One probable interpretation is the existence of alien materials that experienced different irradiation histories from other materials in the Norton County meteorite matrix.
It is interesting to note that the high εSm/εGd samples of the Norton County fractions show high neutron fluences. Figure 4 is a diagram showing the relationship between εSm/εGd and neutron fluences for individual aubrite samples from both this study and previous studies (Hidaka et al. 1999, 2006). The data in the figure can be explained by the two-component mixing between high εSm/εGd with a high neutron fluence component and others. One of the whole rock samples, NCB2, and one of the leachates, NCL#1-2, also show slightly high εSm/εGd values (0.77 and 1.09, respectively), suggesting that these two samples might have been affected by highly irradiated materials. The previously reported εSm/εGd values of Norton County (0.50–0.51) are similar to those recorded for two of the three whole rock samples, NCB1 and NCB2, used in this study. As the LCH model predicts, the εSm/εGd value varies with the chemical composition Σeff, as shown in the figure. However, even considering the differences in Σeff values among individual samples, NCW, NCL#2-2 and NCL#1-2, it is difficult to obtain the high εSm/εGd values predicted by the LCH model.
Figure 4. Diagram showing the relationship between εSm/εGd and neutron fluences for aubritic materials. The data points for closed symbols are in this study. The data points for open symbols are from references (Hidaka et al. 1999, 2006). The data points are classified into two groups: one is a component with high εSm/εGd and high Ψ, and the other is a component with low εSm/εGd and low Ψ.
Download figure:
Standard image High-resolution imageVery high εSm/εGd values of some components in Norton County cannot be explained, as far as we know, only from simple studies and from the Sm and Gd isotopic studies of meteorites and lunar samples, except in the case of Kapoeta. The acid leachates of Kapoeta also show high εSm/εGd values (=1.9), suggesting a different neutron irradiation environment for some components in Kapoeta (Hidaka & Yoneda 2009). As there is a significant amount of solar gas in Kapoeta, some components might have experienced SCR irradiation. Large enrichments of spallation-produced 21Ne and 38Ar in the irradiated grains of Kapoeta are considered to be due to irradiation by energetic proton fluence from an active early (T Tauri) Sun (Caffee et al. 1987). The εSm/εGd values of the leachates from Kapoeta include large analytical uncertainties because of small isotopic shifts of Sm and Gd. On the other hand, the data from Norton County are much clearer, because the isotopic shifts of Sm and Gd are much larger than those of Kapoeta. This enables us to assess interaction with high-energy neutrons.
3.3. REE Characterization
Several attempts have been made to determine the bulk chemical compositions of aubrites. However, aubrites are coarse-grained rocks with minor and trace minerals very heterogeneously distributed throughout the rocks (Wolf et al. 1983). The REE contents of the aubrites provide important information to understand the formation and differentiation process of the meteorite parent body. In order to characterize the probable alien materials having high εSm/εGd and neutron fluence, REE analyses of the individual samples used for the isotopic studies were performed.
The occurrence of some of the exotic sulfides and metallic phases is explained by the formation of aubrites under highly reducing conditions. Among the sulfide minerals in the aubrites, oldhamite (CaS) is a particular material of interest. Oldhamite is known as a major REE carrier in the aubrites (Floss et al. 1990). The variety of REE patterns found in oldhamite from aubrites is considered not to have been produced by a single igneous process (Floss et al. 1990; Floss & Crozaz 1993). Some would have been difficult to produce by igneous processes, but could have been produced by nebular condensates. Furthermore, oldhamite is very refractory; it has a melting point of 2450 ºC and is calculated to be among the first condensates in a reduced solar gas (Larimer 1975; Larimer & Bartholomay 1979; Lodders & Fegley 1993). Since oldhamite is a water-soluble mineral, we planned to chemically separate the oldhamite by the first step of an acid-leaching procedure (see in the description of Section 2.1). The REE contents of individual fractions treated in this study are given in Table 3. The first and second leachates (NCL#1 and #2) of the sequential acid-leaching fractions show higher REE contents than the others (NCL#3, #4, and #5), suggesting the effective dissolution of the oldhamite in the first and second fractions.
Table 3. REE Contents (ppb) of Chemical Separates (NCL#1-1 to NCL#5-2), Mineral Separate (NCW), and Whole Rock (NCB3) of the Norton County Meteorite
La | Ce | Pr | Nd | Sm | Eu | Gd | Tb | Dy | Ho | Er | Tm | Yb | Lu | |
---|---|---|---|---|---|---|---|---|---|---|---|---|---|---|
NCL#1-1 | 354 | 1070 | 184 | 923 | 291 | 24.6 | 363 | 64.5 | 408 | 83.7 | 246 | 31.6 | 191 | 23.8 |
NCL#2-1 | 84.6 | 323 | 56.3 | 273 | 89.1 | 7.39 | 97.5 | 20.0 | 130 | 27.2 | 81.8 | 12.2 | 73.1 | 8.85 |
NCL#3-1 | 4.75 | 16.1 | 2.57 | 12.2 | 3.88 | 0.380 | 4.26 | 0.880 | 5.75 | 1.19 | 3.62 | 0.530 | 3.30 | 0.449 |
NCL#4-1 | 1.39 | 3.32 | 0.413 | 1.75 | 0.437 | 0.0280 | 0.489 | 0.0940 | 0.521 | 0.0980 | 0.317 | 0.0370 | 0.285 | 0.0500 |
NCL#5-1 | 13.1 | 31.1 | 6.00 | 31.6 | 12.1 | 7.70 | 18.2 | 3.79 | 27.2 | 6.60 | 21.9 | 3.40 | 24.6 | 4.59 |
NCL#1-2 | 6.60 | 17.1 | 2.90 | 16.2 | 5.00 | 0.900 | 7.00 | 1.40 | 7.80 | 1.70 | 4.60 | 0.800 | 3.60 | 0.700 |
NCL#2-2 | 4.96 | 16.7 | 2.66 | 13.3 | 4.41 | 0.850 | 5.60 | 1.32 | 8.30 | 1.77 | 5.11 | 0.920 | 4.91 | 0.820 |
NCL#3-2 | 4.67 | 11.3 | 1.11 | 4.01 | 0.956 | 0.0749 | 0.993 | 0.150 | 0.735 | 0.144 | 0.353 | 0.0470 | 0.326 | 0.0453 |
NCL#4-2 | 0.281 | 0.629 | 0.0851 | 0.309 | 0.0977 | 0.0294 | 0.112 | <0.1 | <0.1 | <0.1 | <0.1 | <0.1 | <0.1 | <0.1 |
NCL#5-2 | 2.80 | 8.60 | 1.50 | 8.20 | 3.20 | 1.20 | 4.60 | 1.10 | 6.80 | 1.60 | 4.80 | 0.793 | 5.00 | 1.00 |
NCB3 | 11.8 | 34.0 | 5.44 | 29.3 | 9.78 | 2.34 | 13.3 | 2.74 | 18.2 | 3.88 | 11.9 | 1.85 | 11.3 | 2.07 |
NCW | 4.12 | 11.9 | 1.89 | 9.53 | 4.17 | 0.704 | 5.03 | 1.24 | 8.11 | 1.91 | 6.03 | 1.07 | 6.53 | 1.41 |
Download table as: ASCIITypeset image
The C1-chondrite normalized REE patterns of individual fractions are shown in Figure 5. Norton County shows two different REE patterns in the fractions from individual acid-leaching procedures over two trials. As shown in Figure 5(b), NCL#1-2 and NCL#2-2, from the first and second fractions in the second leaching run, have a relatively flat LREE, and small but clear depletions of Eu and Yb. Similarly, the REE pattern of the NCW phase in Figure 5(b) also has slightly negative anomalies of Eu and Yb. The presence of strong depletions in Eu and Yb in the REE pattern is a property of refractory materials, such as most of the hibonites produced in the early condensation situations (Ireland et al. 1992; Simon et al. 2002; Liu et al. 2009), because Eu and Yb show higher volatilities than the other REEs. Depletions in Eu and Yb imply that the inclusion experienced an intense heating event that allowed these elements to evaporate.
Figure 5. C-1 chondrite-normalized REE patterns of individual samples taken from Norton County. (a) The data of chemical separates from the first leaching run (NCL#1-1 to NCL#5-1); (b) the data of chemical separates from the second leaching run (NCL#1-2 to NCL#5-2), one of whole rock (NCB3), and mineral separate (NCW). The REE patterns of NCL#1-2, NCL#2-2, NCB3, and NCW shown in panel (b) have a depletion of Yb, while those of NCL#1-1 to #5-1 shown in panel (a) do not.
Download figure:
Standard image High-resolution imageOn the other hand, the REE abundance patterns of the leachates NCL#1-1 and NCL#2-1 in Figure 5(a) show relatively flat and negative anomalies of Eu, but no negative anomalies of Yb. The results of the REE contents from two leaching experiments show the heterogeneous distribution of at least two types of oldhamite as an REE carrier. Oldhamite is considered to be analogous to hibonite and perovskite, the two most refractory Ca-bearing phases (Kornacki & Fegley 1986). Therefore, the leachates NCL#1-2 and NCL#2-2 might have predominantly included oldhamite of nebular origin, while the other leachates NCL#1-1 and NCL#2-1 might have predominantly included oldhamite of igneous origin.
Previous REE analyses of oldhamites in aubrites indicate that some grains formed by igneous processes on the parent body, and that others are relict grains that formed during nebular condensation (Floss et al. 1990; Floss & Crozaz 1993; Lodders et al. 1993; Wheelock et al. 1994). The results from our leaching experiments are consistent with the results of previous studies. Besides the concept of the nebula origin of oldhamite, the REE patterns of the leachates NCL #1-2 and NCL#2-2 can be simply explained from the mixing model between early condensates like Group-III hibonite and typical oldhamite without Eu and Yb depletions. A late igneous process might have produced the REE patterns of the mixture having Eu and Yb depletions. REE data also support the possible existence of early condensates from the solar nebula in the Norton County aubrite.
4. CONCLUSIONS
The idea that solar system materials were irradiated by SCR around the early Sun has long been discussed from isotopic evidence of spallogenic nuclides (e.g., Caffee et al. 1987), and been recently renewed from cosmochemical and astronomical viewpoints. The former is shown by isotopic evidence for the existence of 10Be in refractory inclusions of primitive meteorites (McKeegan et al. 2000; Sugiura et al. 2001; MacPherson et al. 2003; Chaussidon et al. 2006; Liu et al. 2009, 2010) because Be is destroyed rather than produced in most stellar events. The latter is from observations of strong X-ray emissions in young stars that are accompanied by intense fluxes of accelerated particles (Feigelson & Montmerle 1999; Feigelson et al. 2002; Güdel 2004).
Solar neutrons are also produced by the interaction of accelerated ions with the solar atmosphere. Considering the making irradiation in the solar nebula, a significant rate of neutron production must also be expected in a similar situation. Materials that originated from the nebula might have experienced irradiation from the early Sun. The high energy of solar neutrons might have gradually decreased the energy during long distance travel, and the solar neutrons might have effectively interacted with 149Sm and 157Gd isotopes of the early condensates in the solar system. The oldhamite of nebular origin is a probable candidate of high-εSm/εGd and high neutron fluence materials in the Norton County meteorite. The REE abundance patterns from the chemical separates also suggest the existence of the early condensates in Norton County.
The 53Cr excess in enstatite chondrites suggests that the enstatite chondrites formed at ∼1.4 AU or even somewhat closer to the Sun (Shukolyukov & Lugmair 2004). Near to the Sun, at a distance of <1 AU, the hydrogen partial pressure would have been high enough to provide the reducing atmosphere. Considering the lithological similarity between aubrites and enstatite chondrites, it is reasonable that the aubrites also formed within ∼1 AU of the Sun (Keil 2010). Since high-energy solar neutrons induced by solar flare events can be detected even on Earth (Debrunner et al. 1993; Watanabe et al. 2003; Sako et al. 2006), such a distance from the Sun is considered adequate for the aubritic materials to sufficiently interact with the solar neutrons produced from the energetic particles from the Sun. Furthermore, the parameter εSm/εGd obtained from the Sm and Gd isotopic structure in this study suggests a higher (harder) energy spectrum (εSm/εGd = 2.1–2.2 at Σeff = 0.0021 shown in Figure 2) of neutrons rather than is characteristic of neutrons induced by GCR (εSm/εGd = 0.4–0.6). Isotopic evidence of a harder neutron energy spectrum was observed in the solar-gas-rich meteorite Kapoeta that experienced early irradiation possibly by the early Sun (Hidaka & Yoneda 2009). In local proton irradiation models (e.g., Lee 1978; Goswami et al. 2001; Gounelle et al. 2001), not many thermal neutrons might have been generated, because the neutrons produced through (p, n) reactions generally have kinetic energies of a few MeV. An appropriate medium is required to efficiently reduce the speed of fast neutrons before they react with 149Sm and 157Gd isotopes. Although the existence of an effective neutron moderator in the early solar system is unclear, the high εSm/εGd values obtained in this study suggest the irradiation of non-thermalized neutrons that insufficiently decreased the energies. It is therefore reasonable to conclude that the data from the separate fractions of the Norton County in this study provide evidence for activity of the early Sun.
The comments and suggestions from the journal editor, Professor E. Feigelson, and the journal reviewer were very useful in revising the first draft of the manuscript. This study was financially supported by a Grant-in-Aid for Scientific Research of the Japan Society for the Promotion of Science (to H.H., No. 21244086).