Abstract
Aim:
Sweroside is an iridoid glycoside with diverse biological activities. In the present study we investigated the effects of sweroside on α-naphthylisothiocyanate (ANIT)-induced cholestatic liver injury in mice.
Methods:
Mice received sweroside (120 mg·kg−1·d−1, ig) or a positive control INT-747 (12 mg·kg−1·d−1, ig) for 5 d, and ANIT (75 mg/kg, ig) was administered on d 3. The mice were euthanized on d 5, and serum biochemical markers, hepatic bile acids and histological changes were analyzed. Hepatic expression of genes related to pro-inflammatory mediators and bile acid metabolism was also assessed. Primary mouse hepatocytes were exposed to a reconstituted mixture of hepatic bile acids, which were markedly elevated in the ANIT-treated mice, and the cell viability and expression of genes related to pro-inflammatory mediators were examined.
Results:
Administration of sweroside or INT-747 effectively ameliorated ANIT-induced cholestatic liver injury in mice, as evidenced by significantly reduced serum biochemical markers and attenuated pathological changes in liver tissues. Furthermore, administration of sweroside or INT-747 significantly decreased ANIT-induced elevation of individual hepatic bile acids, such as β-MCA, CA, and TCA, which were related to its effects on the expression of genes responsible for bile acid synthesis and transport as well as pro-inflammatory responses. Treatment of mouse hepatocytes with the reconstituted bile acid mixture induced significant pro-inflammatory responses without affecting the cell viability.
Conclusion:
Sweroside attenuates ANIT-induced cholestatic liver injury in mice by restoring bile acid synthesis and transport to their normal levels, as well as suppressing pro-inflammatory responses.
Similar content being viewed by others
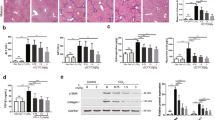
Introduction
Bile acids are the primary constituents of bile and facilitate the absorption of triglycerides, cholesterol and lipid-soluble vitamins. Bile acids play an important role in the enterohepatic system, but the corresponding cytotoxicity to hepatocytes should also be addressed1,2. Numerous studies have suggested that the intrahepatic accumulation of bile acids may directly or indirectly cause further damage to the liver3. One of the hypotheses regarding the pathophysiology of cholestasis is that accumulated hepatic bile acids directly induce cell apoptosis, as supported by studies on rat hepatocytes and human hepatoma lines4,5. Increasing attention has been paid the physiological role of bile acids acting as pro-inflammatory signals, which may trigger hepatic CXC chemokine formation during the development of cholestatic liver injury6,7. The abnormal circulation of bile acids partially reflects liver function8. Therefore, bile acid homeostasis should be well controlled to avoid further liver injury due to their potential hepatotoxicity at high levels.
Cholestasis is an important symptom for liver injury. This condition represents an impairment of the hepatobiliary transport systems, which leads to disruption of circulating bile acid and results in more severe liver damage. Several liver diseases are accompanied by cholestasis, such as gallstones9, hepatitis10, and primary biliary cirrhosis (PBC)11. Therefore, understanding the role of accumulated hepatic bile acids in cholestasic conditions is crucial to elucidate the underlying mechanism of cholestasis. Alpha-naphthylisothiocyanate (ANIT) is a well-characterized cholestatic agent that is widely used to mimic human intrahepatic cholestasis in rodents12,13,14. The biochemical and pathological changes induced by ANIT administration in animal models are similar to the symptoms of clinical intrahepatic cholestasis15. Early studies on the causes of ANIT-induced cholestatic liver injury have emphasized the response of cytotoxic and pro-inflammatory mediators16. Recently, ANIT has been reported to directly induce bile acid overload in hepatocytes, which may partially account for cholestasis17. Therefore, our study investigated the relationship between bile acid homeostasis and inflammatory mediators, which substantially contribute to ANIT-induced cholestatic liver injury.
To date, available drug treatments have proven to be nonspecific and exhibited limited efficacy in cholestasis therapy18,19. Ursodeoxycholic acid is the only drug approved by the Food and Drug Administration for cholestasis treatment20,21. INT-747 has been investigated in a phase II trial for the treatment of PBC, liver fibrosis, and diet-induced atherosclerosis22. However, side effects of INT-747 on lipid metabolism have been observed in pre-clinical studies, and these side effects may limit drug development. Therefore, the development of novel therapies for cholestasis is urgently needed.
Numerous studies have investigated the use of natural phytochemicals for the prevention and treatment of cholestasis. Sweroside is an iridoid glycoside that exhibits diverse biological activities, such as anti-fungal, anti-diabetic, anti-inflammatory, and anti-tumor effects23,24,25,26. Many studies have indicated that sweroside ameliorates liver injury by decreasing oxidative damage and inhibiting the production of pro-inflammatory kinases27,28. However, the effects of sweroside on mice with intrahepatic cholestasis is not clear. The current study performed bile acid profile analyses to evaluate the hepatoprotective effects of sweroside. The expression levels of bile acid metabolism-related genes and pro-inflammatory kinases were measured to identify the underlying mechanism. Subsequently, we determined whether sweroside ameliorated ANIT-induced cholestatic liver injury by regulating bile acids and further mediating pro-inflammatory responses.
Materials and methods
Materials
Sweroside (>98.5% purity) was purchased from NanJing Goren Biotechnology Co Ltd (Nanjing, China). INT-747 was purchased from Adipogen (San Diego, CA, USA). ANIT and olive oil were purchased from Sigma-Aldrich (St Louis, MO, USA). All bile acid standards were purchased from Steraloids (Rhode Island, USA) and Sigma-Aldrich. Ammonium acetate, formic acid, methanol (MeOH), and acetonitrile (HPLC-grade) were purchased from Fisher Scientific (Nepean, Ontario, Canada). Ultrapure water was purified with a Milli-Q system (Millipore, Bedfordshire, MA, USA). Other solvents of analytical grade were purchased from Shanghai Chemical Factory (Shanghai, China). Dulbecco's modified Eagle's medium (DMEM), fetal bovine serum (FBS), L-glutamine, and penicillin–streptomycin were purchased from Fisher Scientific. Non-essential amino acids, insulin, and hydrocortisone were purchased from Sigma-Aldrich.
Ethics statement
The Guide for the Care and Use of Laboratory Animals was strictly followed, and the Institutional Animal Committee of Shanghai University of Traditional Chinese Medicine approved the animal experiment protocols (Permit number: SCXK (Hu) 2012-0002).
In vivo study
C57BL/6J mice (pathogen free, 25–30 g, 8–10 weeks of age) were obtained from the Laboratory Animal Center of Shanghai University of Traditional Chinese Medicine. The animals were housed at 20±2 °C with 60%–70% relative humidity under a 12-h light/dark cycle. Mice were provided food and tap water ad libitum. The mice were randomly assigned into four groups: Vehicle group (n=8), ANIT group (n=8), Sweroside+ANIT group (n=8), and INT-747+ANIT group (n=8). INT-747 has been used for cholestasis treatment because it activates the FXR. INT-747 was used as the positive control in the pharmacological experiments in the present study. Sweroside+ANIT and INT-747+ANIT groups were treated with sweroside (120 mg/kg, ig) or INT-747 (12 mg/kg, ig) for five continuous days (once daily). The Vehicle group and ANIT group received normal saline. ANIT was administered to the ANIT, Sweroside+ANIT and INT-747+ANIT groups on the 3rd day, and this was followed by normal saline, sweroside or INT-747 treatment 4 h later. Forty-eighty hours after ANIT administration, all mice were euthanized by CO2 inhalation after having fasted for 12 h. Retro-orbital blood samples were collected into tubes, and this was followed by centrifugation at 4 °C for 10 min (8000×g). Each mouse liver was dissected, and a portion was fixed in 10% buffered formaldehyde for further histological analyses. The remaining liver samples were immediately transferred to liquid nitrogen and stored at -80 °C for real-time PCR.
Serum biochemistry and hepatic myeloperoxidase assay
The serum levels of aspartate aminotransferase (AST), alanine aminotransferase (ALT), alkaline phosphatase (ALP), total bilirubin (TBIL), direct bilirubin (DBIL), and total bile acids (TBA) were determined with commercially available kits in an automatic biochemical analyzer (HITACHI 7080, Japan). Hepatic myeloperoxidase (MPO) activity was determined with a commercial assay kit according to the manufacturer's protocol (Nanjing Jiancheng Bioengineering Institute, Nanjing, China).
Histological analysis
Liver samples from all groups were fixed in 10% formaldehyde (neutral buffered formalin), dehydrated, embedded in paraffin, cut into 5-μm sections, and stained with hematoxylin and eosin (H&E) according to standard protocols. Shanghai Showbio Biotech, Inc performed histological assessments.
Quantification of bile acids
Our bile acid quantification method was used and improved on the basis of our previous study. Briefly, approximately 50 mg of liver was homogenized in 2 volumes of 50% MeOH. Liver homogenate (300 μL) was spiked with 2 mL of ice-cold MeOH, vortexed, was shaken continuously for 1 h, and was centrifuged at 12 000×g for 10 min. The supernatant was aspirated, and the precipitate was extracted with another 1 mL of ice-cold MeOH. Supernatants from the two extraction steps were pooled and evaporated to dryness, and this was followed by reconstitution in a 100-μL mixture of 0.1% formic acid containing 5 mmol/L ammonium acetate–methanol (45:55, v/v) prior to analysis. The sample solution was centrifuged at 12 000×g for 10 min at 4 °C, and a 5-μL aliquot was injected for UPLC/MS analysis.
Bile acids were determined with a Waters Acquity ultra-performance lipid chromatograph system (Waters, MA, USA) equipped with an Acquity UPLC BEH C18 column (1.7 μm, 2.1 mm×100 mm, Waters) at 45 °C. The mobile phase consisted of 0.1% formic acid in an aqueous solution of 5 mmol/L ammonium acetate (A) and methanol (B) at a flow rate of 0.3 mL/min. The elution gradient was performed as follows. The eluent composition was set at 55% A and 45% B for the first minute, linearly changed to 62% A and 38% B at 2.6 min, and the proportion of B was increased to 80% in the next 8.8 min. The sample injection volume was 5 μL.
MS analysis was performed with a ZQ 2000 quadrupole spectrometer equipped with an ESI probe and operated with selective ion monitoring in the negative-ion mode (Waters, MA, USA). The capillary and cone voltages were set at 3.0 and 55 V, respectively. The source temperature was 120 °C, and the desolvation temperature was 300 °C. The desolvation gas flow was set at 700 L/h, and the cone gas flow rate was set at 50 L/h. Data were acquired and processed with MassLynx 4.1 software.
In vitro study
Primary hepatocytes of male C57BL6 mice were purchased from the Research Institute for Liver Disease (Shanghai) Co, Ltd. Primary hepatocytes were cultured in DMEM supplemented with 10% fetal bovine serum, L-glutamine (2 mmol/L), non-essential amino acids (2 mmol/L), insulin (0.08 IU/mL), hydrocortisone (2.34 μmol/L), and penicillin–streptomycin (1%). Cells were treated with the reconstituted bile acid mixture, which was composed of the hepatic bile acids that were most elevated after ANIT exposure in vivo. Cell viability was assessed with a CCK-8 assay kit (Shanghai Yeasen Biotechnology, Shanghai, China). The gene expression of pre-inflammatory mediators was also examined.
RNA isolation and quantitative real-time PCR
Total RNA was isolated from mouse liver samples and mouse primary hepatocytes with Trizol (Takara, Dalian, China) according to the manufacturer's protocol. RNA was reverse transcribed into cDNA (1 μg/μL) with a PrimerScript RT Reagent Kit (Takara, Dalian, China). Gene-specific primers were designed online with Primers 3. Table 1 presents the primers for the detected genes. Quantification of gene expression was performed with real-time PCR (ABI, USA) with SYBR Green Master Mix (Takara, Dalian, China). All reactions were performed in triplicate with the following thermal cycling conditions: initial denaturation at 95 °C for 30 s followed by 50 cycles of 95 °C for 5 s and 60 °C for 30 s. Beta-actin was used as an internal reference to calculate the relative expression of target genes.
ELISA assay
Protein levels of TNF-α, IL-6, MIP-2, and ICAM-1 were determined in all treated groups by using commercial kits (Shanghai Xinyu Biotechnology Company, Shanghai, China).
Statistical analysis
Data were statistically analyzed by one-way ANOVA and the least significant differences test (SPSS 18.0 software; SPSS, Inc, Chicago, USA). Differences were considered to be statistically significant when P<0.05. OPLS-DA analysis was performed with Simca-P software 13.0 (Umetrics, Umea, Sweden). All graphs were generated with Graphpad Prism 5 (GraphPad Software, CA, USA).
Results
Sweroside exhibited hepatoprotective effects against ANIT-induced cholestasis
Biochemical indicators were detected in all treatment groups to evaluate the hepatoprotective effects of sweroside. ANIT administration significantly increased serum ALT and AST in mice. However, sweroside treatment dramatically reduced both of these indicators. Sweroside treatment also reversed the ANIT-induced increases in serum ALP, TBIL, DBIL, and TBA, all of which indicated biliary toxicity. Oral INT-747 administration markedly decreased serum AST, ALP, TBIL, DBIL, and TBA levels but only slightly decreased ALT (Figure 1A). Liver sections were stained with H&E to further examine histological changes in the liver after ANIT exposure. Multifocal hepatic parenchymal necrosis with inflammatory cell infiltration was observed in the ANIT-treated group. Sweroside and INT-747 treatment significantly ameliorated the degree of hepatic parenchymal necrosis and attenuated inflammatory cell infiltration (Figure 1B).
Sweroside alleviates ANIT-induced hepatotoxicity and cholestasis. (A) Biochemical indicators in mice orally administered sweroside (120 mg/kg, ig) or INT-747 (12 mg/kg, ig) for five consecutive days (once daily) were determined 48 h after vehicle or ANIT exposure. All data are shown as the mean±SEM (n=8). nsP>0.05, *P<0.05, **P<0.01 versus vehicle group. nsP>0.05, #P<0.05, ##P<0.01 versus ANIT group. (B) The representative images of H&E-stained liver sections (100× magnification) were examined under a microscope.
Hepatoprotective effects of sweroside against ANIT-induced cholestasis were related to the regulation of bile acid homeostasis
Hepatic bile acid overload is a primary characteristic of ANIT-induced cholestasis. Figure 2A shows that ANIT administration markedly increased hepatic TBA levels. Individual hepatic bile acid levels were measured by UPLC–MS and followed by OPLS-DA analysis. Figure 2B shows that the vehicle, ANIT, Sweroside+ANIT, and INT-747+ANIT groups were markedly separated from each other in OPLS-DA score plots. The ANIT group was scattered far from the vehicle group, thereby indicating a severe disruption of bile acid homeostasis after ANIT administration. The sweroside pre-treatment group was closer to the vehicle group than to the ANIT group, thereby indicating that sweroside normalized the ANIT-induced abnormality of hepatic bile acid levels. The INT-747 treatment group exhibited an identical trend to that of the sweroside group. Figure 2C shows that ANIT exposure markedly increased hepatic β-MCA, ω-MCA, CA, T-ω-MCA, TCDCA, TDCA, and TCA levels. However, oral sweroside or INT-747 administration significantly reduced the hepatic accumulation of the above-mentioned bile acids, especially the reduction of TCA. Sweroside restored bile acid profiles to normal after ANIT exposure, thereby suggesting that bile acid profiles are good markers to evaluate the protective effects of sweroside.
Sweroside alters hepatic bile acid profiles and protects livers against ANIT-induced cholestatic liver injury. (A) Total bile acid content was detected in the liver. (B) OPLS-DA score plots derived from the hepatic bile acid levels of the vehicle, ANIT, Sweroside+ANIT, and INT-747+ANIT groups. (C) Concentrations of individual bile acids were detected in the liver. All data are shown as the mean±SEM (n=8). nsP>0.05, *P<0.05, **P<0.01 versus vehicle group. nsP>0.05, #P<0.05, ##P<0.01 versus ANIT group.
Sweroside mediated bile acid homeostasis against ANIT-induced cholestasis by affecting bile acid synthesis and transport
The mRNA levels of major bile acid synthesis enzymes and transporters were measured to elucidate the protective mechanisms of sweroside on bile acid regulation. Figure 3 shows that ANIT exposure significantly down-regulated most bile acid biosynthesis genes (Cyp7a1, Cyp8b1, Cyp27a1, Cyp7b1, Hsd3b7, and Akr1d1). However, sweroside treatment significantly reversed the ANIT-induced down-regulation of Cyp7a1, Cyp8b1, and Hsd3b7 but further decreased the down-regulation of Cyp7b1 (Figure 3). In contrast, INT-747 treatment, compared with ANIT treatment, further decreased the down-regulation of Cyp7a1 and Cyp8b1 and resulted in increased expression of Cyp27a1 and Cyp7b1 . However, INT-747 had no effect on Hsd3b7 and Akr1d1 expression levels. Efflux transporters were also studied, including Ost-β, Mrp2, Bsep, and Mdr1. ANIT exposure significantly increased the expression of Ost-β and Mdr1, but Bsep expression was only slightly increased compared with the vehicle group. ANIT exposure significantly decreased the expression of Mrp2 mRNA. Sweroside treatment reversed the up-regulated Ost-β and Mdr1 mRNA expression and slightly increased the up-regulation of Bsep mRNA, as compared with ANIT group. However, INT-747 treatment significantly reversed Mrp2 and Ost-β expression and slightly enhanced the up-regulation of Bsep and Mrd1 expression. Ntcp, Oatp1a1, Oatp1a4, and Oatp1b2 play critical roles in the control of bile acid uptake, and ANIT exposure significantly decreased the expression of these mRNAs. Figure 4 shows that sweroside treatment enhanced the down-regulation of Ntcp, Oatp1a1, and Oatp1b2, but it did not affect the Oatp1a4 mRNA level, as compared with the ANIT group. In contrast, INT-747 increased the Oatp1b2 expression level, as compared with the ANIT group. The expression of Ntcp, Oatp1a1, and Oatp1a4 remained unchanged after INT-747 treatment.
Sweroside alters the gene expression profiles involved in bile acid synthesis and transport. Quantitative real-time PCR analysis was performed to measure the gene expression of Cyp7a1, Cyp8b1, Cyp27a1, Cyp7b1, Akr1d1, Hsd3b7, Oatp1a1, Oatp1a4, Oatp1b2, Ntcp, Ost-β, Mrp2, Bsep, and Mdr1 in the liver. All data are shown as the mean±SEM (n=8). nsP>0.05, *P<0.05, **P<0.01 versus vehicle group. nsP>0.05, #P<0.05, ##P<0.01 versus ANIT group.
Sweroside decreases hepatic MPO activity and represses the pro-inflammatory response. (A) Hepatic MPO activity was assayed. (B) Quantitative real-time PCR analysis was performed to measure the gene expression of TNF-α, IL-6, mKC, MIP-2, and ICAM-1 in the liver. (C) Commercially available ELISA test kits were used to determine the protein expression levels of IL-6, TNF-α, ICAM-1, and MIP-2. All data are shown as the mean±SEM (n=8). nsP>0.05, *P<0.05, **P<0.01 versus vehicle group. nsP>0.05, #P<0.05, ##P<0.01 versus ANIT group.
Sweroside repressed pro-inflammatory responses against ANIT-induced cholestatic liver injury
Most studies have suggested that inflammatory responses are histological features of cholestasis-associated pathophysiological changes. Our results demonstrated that hepatic MPO activity and the gene expression levels of TNF-α, IL-6, mKC, MIP-2, and ICAM-1 increased significantly in mice after ANIT exposure (Figure 4A and 4B). However, oral sweroside administration significantly ameliorated hepatic MPO activity and TNF-α, IL-6, mKC, MIP-2, and ICAM-1 levels, thereby indicating that sweroside exerts potential anti-inflammatory effects. INT-747 slightly decreased hepatic MPO activity and significantly ameliorated ANIT-induced increased mRNA levels of TNF-α, IL-6, mKC, MIP-2, and ICAM-1 in our study (Figure 4A and 4B). Sweroside and INT-747 also decreased the ANIT-induced elevated protein levels of TNF-α, IL-6, MIP-2, and ICAM-1 in sera (Figure 4C).
Hepatic accumulation of bile acids induced pro-inflammatory responses
Primary hepatocytes in male C57BL6 mice were treated with a reconstituted bile acid mixture to investigate whether increased hepatic concentrations of bile acids after ANIT exposure were required for cell death or the release of pro-inflammatory mediators. Figure 2 shows the exact composition of the bile acid mixture. Treatment with the reconstituted bile acid mixture produced no significant cell death induction, which was supported by the cell viability study (Figure 5A). However, the reconstituted bile acids markedly increased TNF-α, IL-6, IL-17α, mKC, MIP-2, and ICAM-1 mRNA expression levels (Figure 5B). These results are consistent with the results after ANIT administration in vivo. Our data indicate that bile acids may induce the inflammatory mediators that contribute to cholestasis.
Cell viability study and expression of pro-inflammatory cytokines after exposure to the bile acid mixture. Primary mouse hepatocytes were treated with the indicated concentration of bile acid mixture. (A) Cell viability study using a CCK-8 kit. (B) Real-time PCR was used to assess the gene expression of TNF-α, IL-6, IL-17α, mKC, MIP-2, and ICAM-1. All data are shown as the mean±SEM (n=8). **P<0.01 versus control group.
Discussion
This study assessed the hepatoprotective effect of sweroside by using an ANIT-induced cholestasis model and bile acid profile analysis. Many reports have focused on the beneficial role of sweroside24,25,26,27,28, but its effects on cholestatic liver injury remain largely unknown.
Sweroside exhibited the most dramatically protective effect at a dose of 120 mg/kg in our preliminary dose-response study, as evidenced by the reduction of ALT, AST, ALP, TBA, TBIL, and DBIL (Supplementary Figure S1). Therefore, this dose of sweroside (120 mg/kg) was chosen for the current study of the underlying mechanism. Our results demonstrated that the potential hepatoprotective benefits of sweroside against ANIT-induced liver injury occurred via bile acid regulation. We also discovered that sweroside significantly decreased TBA and TG levels in normal mice (Supplementary Figure S2 and Supplementary Figure S3B), thus partially suggesting its protective effect in the ANIT-induced model. An abnormal accumulation of bile acids in hepatocytes has been observed in cholestatic diseases, including intrahepatic cholestasis of pregnancy and progressive familial intrahepatic cholestasis 1, 2, and 3 (PFIC1, PFIC2, and PFIC3)29,30,31. Therefore, maintaining the homeostasis of bile acids plays an importance role in cholestasis prevention and therapy. ANIT exposure significantly increased hepatic TBA and individual bile acids in the present study, which is consistent with results from a previous report32. Sweroside demonstrated potential hepatoprotective effects through alleviation of bile acid accumulation in the liver. Bile acid metabolism-related genes warrant further study to investigate the mechanism underlying the roles of sweroside in bile acid regulation in an animal model.
Previous reports have demonstrated that bile acid synthesis may be inhibited during liver damage33,34. The present study demonstrated that sweroside significantly attenuated the down-regulation of Cyp7a1, Cyp8b1, and Hsd3b7, which is consistent with results from previous reports14,17. INT-747 further decreased the mRNA expression of Cyp7a1 and Cyp8b1 but reversed the Cyp7b1 expression, as compared with the ANIT group. We hypothesize that sweroside affects the de novo synthesis of bile acids in a unique manner, which differs from that of INT-747. A previous report has demonstrated that ANIT also affects the function of bile acid transporters, ranging from efflux transporters to uptake transporters35. ANIT significantly decreased Mrp2, Ntcp, Oatp1a1, Oatp1a4, and Oatp1b2 levels and increased Ost-β and Mdr1 levels in our study. These data are consistent with a results from a previous report36. However, sweroside attenuated ANIT-induced changes in Mrp2, Ost-β, Mdr1, Ntcp, Oatp1a1, and Oatp1b2 expression, thereby restoring the disruption of bile acid profile. Mrp2 is an efflux transporter that mediates the secretion of toxic endogenous and exogenous compounds in the form of amphiphilic anionic conjugates via an ATP-dependent mechanism37,38. Sweroside treatment enhanced Mrp2 expression, which may have contributed to the accelerated secretion of hepatic bile acids. Serum bilirubin is a substrate of Mrp2, and this factor negatively correlated with Mrp2 expression. GSH is another substrate of Mrp2, which contributed to the further attenuation of the ANIT-induced liver injury by competitively inhibiting the conjugation between ANIT and GSH. Ost-β is also an important bile acid efflux gene that transports bile acids into the bloodstream. ANIT exposure robustly increased Ost-β expression, a result consistent with those from a previous report39. The reduced expression of Ost-β by sweroside may contribute to the decreased counter-transport of bile acids into the blood, which further reduces bile acid levels in the systemic circulation. Ntcp and Oatp are located at the basolateral surface of hepatocytes and are responsible for the hepatic uptake of bile acids. A reduction of Ntcp has been observed in various cholestatic conditions, such as advanced PBC, cholestatic alcoholic liver injury, and biliary atresia40. Therefore, the restoration of defective Ntcp in cholestasis may be an important target for pharmacotherapeutic interventions. In our previous study, the Danning tablet was used to treat cholestatic liver injury, and this treatment partially exerts its protective effect via the restoration of Ntcp expression13. Oatp1a1 that has a high affinity for TCA and TDCA is another gene involved in the hepatic uptake of bile acids41. The up-regulation of Oatp1a1 by sweroside treatment in the present study is consistent with the reduction of TCA and TDCA in the liver.
The modulation of pro-inflammatory responses plays a critical role in cholestatic liver injury33. The present study demonstrated that hepatic MPO activity was significantly increased after ANIT exposure, which is consistent with results from a previous study34. However, oral sweroside administration alleviated the increase in hepatic MPO activity after ANIT treatment, which contributed to the inhibition of neutrophil infiltration into liver tissues. Sweroside also exhibits anti-inflammatory activity by inhibition of NO, IL-6, and TNF-α production induced by lipopolysaccharide (LPS) in RAW264 cells29. Sweroside decreased hepatic mRNA and protein expression of pro-inflammatory cytokines in the present study, including TNF-α, IL-6, mKC, MIP-2, and ICAM-1 (Figure 4B and 4C). The relationship between pro-inflammatory cytokines and bile acid metabolism-related genes has recently received considerable attention. IL-1β has been identified as an inducer of Mrp2, Cyp8b1, and Cyp7a142,43,44. TNF-α mediates the down-regulation of the Oatp genes at the mRNA level45. Mrp2, Cyp8b1, Cyp7a1 and Oatp expression was negatively correlated with the pro-inflammatory cytokine expression in our study, which indicates that the suppression of bile acid metabolism-related genes may be partially caused by an elevation of the pro-inflammatory response. However, further studies are necessary to verify this hypothesis.
ANIT induces pro-inflammatory mediators16. However, the role of increased hepatic bile acids in the progress of ANIT-induced liver injury is not known. A bile acid mixture significantly increased the mRNA expression of pro-inflammatory cytokines in vitro in our study, which is consistent with results from a previous report5. TCA exhibited the highest increase after ANIT treatment in our study, and this factor increases the expression of MIP-2 in rodents and IL-8 in humans6,7. The reconstituted bile acid mixture markedly increased mKC and MIP-2 expression in vitro in our study, which is consistent with the results after ANIT administration in vivo. Therefore, bile acids may act as a pro-inflammatory signal triggering CXC chemokine formation and result in liver injury.
Sweroside treatment of cells or mice exhibited no obvious toxicity (Supplementary Figure S3 and Supplementary Figure S4), thus supporting the safety of sweroside as a potentially reliable treatment option for further consideration. In conclusion, sweroside exerted a protective effect on ANIT-induced acute cholestatic liver injury in mice. Target profiling analysis of individual hepatic bile acids was used to evaluate the protective effect of sweroside against liver injury. The anti-inflammatory activity of sweroside also prevented ANIT-induced acute cholestatic liver injury. These combined results indicate that the hepatoprotective mechanism of sweroside is related to the regulation of bile acid homeostasis, and abnormal bile acid levels may result in pro-inflammatory responses that lead to liver injury.
Abbreviations
ANIT, a-naphthylisothiocyanate; INT-747, obeticholic acid; mKC, mouse keratinocyte derived factor; MIP-2, macrophage inflammatory protein 2; ICAM-1, intercellular adhesion molecule-1; TNF-α, tumor necrosis factor α; IL-6, interleukin-6; IL-17α, interleukin 17α; TBA, total bile acid; α-MCA, α-muricholic acid; β-MCA, β-muricholic acid; ω-MCA, ω-muricholic acid; T-α-MCA, tauro-α-muricholic acid; T-β-MCA, tauro-β-muricholic acid; T-ω-MCA, tauro-ω-muricholic acid; CA, cholic acid; DCA, deoxycholic acid; CDCA, chenocholic acid; LCA, lithocholic acid; UDCA, ursodeoxycholic acid; HDCA, hyodesoxycholic acid; GCA, glycocholicacid; TCA, taurocholic acid; GDCA, glycodeoxycholic; TDCA, taurodeoxycholic acid; GCDCA, glycochenodeoxycholic acid; TCDCA, taurochenodeoxycholic acid; GUDCA, glycoursodeoxycholic acid; TUDCA, tauroursodeoxycholic acid; THDCA, taurohyodesoxycholic acid; GLCA, glycolithocholic acid; TLCA, taurolithocholic acid.
Author contribution
Qiao-ling YANG, Li YANG, and Zheng-tao WANG conceived and designed the experiments; Qiao-ling YANG, Jun-ting GONG, Xiao-wen TANG, and Guang-yun WANG performed the experiments; Qiao-ling YANG, Jun-ting GONG, Xiao-wen TANG, and Guang-yun WANG acquired data; Qiao-ling YANG and Fan YANG analyzed data; Qiao-ling YANG, Fan YANG, and Li YANG wrote the manuscript; all authors revised the manuscript.
References
Wollbright BL, Li F, Xie Y, Farhood A, Fickert P, Trauner M, et al. Lithocholic acid feeding results in direct hepato-toxicity independent of neutrophil function in mice. Toxicol Lett 2014; 228: 56–66.
Chatterjee S, Bijsmans IT, van Mil SW, Augustijns P, Annaert P . Toxicity and intracellular accumulation of bile acids in sandwich-cultured rat hepatocytes: role of glycine conjugates. Toxicol In Vitro 2014; 28: 218–30.
Malhi H, Guicciardi ME, Gores GJ . Hepatocyte death: a clear and present danger. Physiol Rev 2010; 90: 1165–94.
Faubion WA, Guicciardi ME, Miyoshi H, Bronk SF, Roberts PJ, Svingen PA, et al. Toxic bile salts induce rodent hepatocyte apoptosis via direct activation of Fas. J Clin Invest 1999; 103: 137–45.
Woolbright BL, Dorko K, Antoine DJ, Clarke JI, Gholami P, Li F, et al. Bile acid-induced necrosis in primary human hepatocytes and in patients with obstructive cholestasis. Toxicol Appl Pharmacol 2015; 283: 168–77.
Allen K, Jaeschke H, Copple BL . Bile acids induce inflammatory genes in hepatocytes: a novel mechanism of inflammation during obstructive cholestasis. Am J Pathol 2011; 178: 175–86.
O'Brien KM, Allen KM, Rockwell CE, Towery K, Luyendyk JP, Copple BL . IL-17α synergistically enhances bile acid-induced inflammation during obstructive cholestasis. Am J Pathol 2013; 183: 1498–507.
Paumgartner G . Medical treatment of cholestatic liver diseases: from pathobiology to pharmacological targets. World J Gastroenterol 2006; 12: 4445–51.
Ji F, Deng H, Li Z . Eltrombopag for thrombocytopenic patients with hepatitis C virus infection and cirrhosis. Gastroenterology 2014; 147: 253–4.
Wang L, Wang J, Shi Y, Zhou X, Wang X, Li Z, et al. Identification of a primary biliary cirrhosis associated protein as lysosome-associated membrane protein-2. J Proteomics 2013; 91: 569–79.
Hirschfield GM, Chapman R, Karlsen TH, Lammert F, Lazaridis KN, Mason AL . The genetics of complex cholestatic disorders. Gastroenterology 2013; 144: 1357–74.
Yang F, Xu Y, Xiong A, He Y, Yang L, Wang Z . Evaluation of the protctive effect of Rhei Radix et Rhizoma against α-naphthylisothiocyanate induced liver injury based on metabolic profile of bile acids. J Ethnopharmacol 2012; 18; 144: 599–60.
Ding L, Zhang B, Zhan C, Yang L, Wang Z . Danning tablets attenuates α-naphthylisothiocyanate induced cholestasis by modulating the expression of transporters and metabolic enzymes. BMC Complement Altern Med 2014; 14: 249.
Yan JY, Ai G, Zhang XJ, Xu HJ, Huang ZM . Investigations of the total flavonoids extracted from flowers of Abelmoschus manihot (L) Medic against α-naphthylisothiocyanate-induced cholestatic liver injury in rats. J Ethnopharmacol 2015; 172: 202–13.
Erlinger S . What is cholestasis in 1985? J Hepatol 1985; 1: 687–93.
Kodali P, Wu P, Lahiji PA, Brown EJ, Maher JJ . ANIT toxicity toward mouse hepatocytes in vivo is mediated primarily by neutrophils via CD18. Am J Physiol Gastrointest Liver Physiol 2006; 291: G355–63.
Guo C, He L, Yao D, A J, Cao B, Ren J, et al. Alpha-naphthylisothiocyanate modulates hepatobiliary transporters in sandwich-cultured rat hepatocyte. Toxicol Lett 2014; 224: 93–100.
Choi Y, Yi NJ, Ko JS, Ko JM, Jin US, Kim HS, et al. Living donor liver transplantation for an infant with osteogenesis imperfecta and intrahepatic cholestasis: report of a case. J Korean Med Sci 2014; 29: 441–4.
Hirschfield GM, Heathcote EJ, Gershwin ME . Pathogenesis of cholestatic liver disease and therapeutic approaches. Gastroenterology 2010; 139: 1481–96.
Beuers U, Trauner M, Jansen P, Poupon R . New paradigms in the treatment of hepatic cholestasis: from UDCA to FXR, PXR and beyond. J Hepatol 2015; 62: S25–37.
Fickert P, Pollheimer MJ, Silbert D, Moustafa T, Halilbasic E, Krones E, et al. Differential effects of nor UDCA and UDCA in obstructive cholestasis in mice. J Hepatol 2013; 58: 1201–8.
Fiorucci S, Cipriani S, Mencarelli A, Baldelli F, Bifulco G, Zampella A . Farnesoid X receptor agonist for the treatment of liver and metabolic disorders: focus on 6-ethyl-CDCA. Mini Rev Med Chem 2011; 11: 753–62.
Tan RX, Wolfender JL, Ma WG, Zhang LX, Hostettmann K . Secoiridoids and anti-fungal aromatic acids from Gentiana algida. Phytochemistry 1996; 41: 111–6.
Hase K, Li JX, Basnet P, Xiong Q, Takamura SC, Namba T, et al. Hepatoprotective principles of Swertia japonica Makino on D-galactosamine/lipopolysaccharide-induced liver injury in mice. Chem Pharm Bull 1997; 45: 1823–7.
Mahendran G, Thamotharan G, Sengottuvelu S, Narmtha Bai V . Anti-diabetic activity of Swertia corymbosa (Griseb.) Wight ex C.B. Clarke aerial parts extract in streptozotocin induced diabetic rats. J Ethnopharmacol 2014; 151: 1175–83.
Sun H, Li L, Zhang A, Zhang N, Lv H, Sun W, et al. Protective effects of sweroside on human MG-63 cells and rat osteoblasts. Fitoterapia 2013; 84: 174–9.
Jeong YT, Jeong SC, Hwang JS, Kim JH . Modulation effects of sweroside isolated from the Lonicera japonica on melanin synthesis. Chem Biol Interact 2015; 238: 33–9.
He YM, Zhu S, Ge YW, Kazuma K, Zou K, Cai SQ, et al. The anti-inflammatory secoiridoid glycosides from Gentianae Scabrae Radix: the root and rhizome of Gentiana scabra. J Nat Med 2015; 69: 303–12.
Chen F, Ananthanarayanan M, Emre S, Neimark E, Bull LN, Knisely AS, et al. Progressive familial intrahepatic cholestasis, type 1, is associated with decreased farnesoid X receptor activity. Gastroenterology 2004; 126: 756–64.
Strautnieks SS, Bull LN, Knisely AS, Kocoshis SA, Dahl N, Arnell H, et al. A gene encoding a liver-specific ABC transporter is mutated in progressive familial intrahepatic cholestasis. Nat Genet 1998; 20: 233–8.
van Mil SWC, van der Woerd WL, van der Brugge G, Sturm E, Jansen PLM, Bull LN, et al. Benign recurrent intrahepatic cholestasis type 2 is caused by mutations in ABCB11. Gastroenterology 2004; 127: 379–84.
Cui YJ, Aleksunes LM, Tanaka Y, Goedken MJ, Klaassen CD . Compensatory induction of liver efflux transporters in response to ANIT-induced liver injury is impaired in FXR-null mice. Toxicol Sci 2009; 110: 47–60.
Thatch KA, Schwartz MZ, Yoo EY, Mendelson KG, Duke DS . Modulation of the inflammatory response and apoptosis using epidermal growth factor and hepatocyte growth factor in a liver injury model: a potential approach to the management and treatment of cholestatic liver disease. J Pediatr Surg 2008; 43: 2169–73.
Wang T, Zhou ZX, Sun LX, Li X, Xu ZM, Chen M, et al. Resveratrol effectively attenuates α-naphthyl-isothiocyanate-induced acute cholestasis and liver injury through choleretic and anti-inflammatory mechanisms. Acta Pharmacol Sin 2014; 35: 1527–36.
Liu Y, Binz J, Numerick MJ, Dennis S, Luo G, Desai B, et al. Hepatoprotection by the farnesoid X receptor agonist GW4064 in rat models of intrahepatic and extrahepatic cholestasis. J Clin Invest 2003; 112: 1878–87.
Guo C, He L, Yao D, A J, Cao B, Ren J, et al. Alpha-naphthylisothiocyanate modulates hepatobiliary transporters in sandwich-cultured rat hepatocytes. Toxicol Lett 2014; 224: 93–100.
Keppler D . Multidrug resistance proteins (MRPs, ABCCs): importance for pathophysiology and drug therapy. Handb Exp Pharmacol 2011; 201: 299–323.
Dietrich CG, Ottenhoff R, de Waart DR, Oude Elferink RPJ . Role of Mrp2 and GSH in intrahepatic cycling of toxins. Toxicology 2001; 167: 73–81.
Boyer JL, Trauner M, Mennone A, Soroka CJ, Cai SY, Moustafa T, et al. Up-regulation of a basolateral FXR-dependent bile acid efflux transporter OST alpha-OST beta in cholestasis in humans and rodents. Am J Physiol Gastrointest Liver Physiol 2006; 290: G1124–30.
Zollner G, Fickert P, Zenz R, Fuchsbichler A, Stumptner C, Kenner L, et al. Hepatobiliary transporter expression in percutaneous liver biopsies of patients with cholestatic liver diseases. Hepatology 2001; 33: 633–46.
Zhang Y, Csanaky IL, Lehman-McKeeman LD, Klaassen CD . Loss of organic anion transporting polypeptide 1a1 increases deoxycholic acid absorption in mice by increasing intestinal permeability. Toxicol Sci 2011; 124: 251–60.
Denson LA, Bohan A, Held MA, Boyer JL . Organ-specific alterations in RAR alpha: RXR alpha abundance regulate rat Mrp2 (Abcc2) expression in obstructive cholestasis. Gastroenterology 2002; 123: 599–607.
Jahan A, Chiang JY . Cytokine regulation of human sterol 12alpha-hydroxylase (CYP8B1) gene. Am J Physiol Gastrointest Liver Physiol 2005; 288: G685–95.
Li T, Jahan A, Chiang JY . Bile acids and cytokines inhibit the human cholesterol 7 alpha-hydroxylase gene via the JNK/c-jun pathway in human liver cells. Hepatology 2006; 43: 1202–10.
Geier A, Dietrich CG, Trauner M, Gartung C . Extrahepatic cholestasis down-regulates Oatp1 by TNF-alpha signaling without affecting Oatp2 and Oatp4 expression and sodium-independent bile salt uptake in rat liver. Liver Int 2007; 27: 1056–65.
Acknowledgements
This work was financially supported by the Natural Science Foundations of China (81222053 and 81573581), the Program for New Century Excellent Talents in University (NCET-12-1056) and the National S&T Major Special Projects (2014ZX09301306-007).
Author information
Authors and Affiliations
Corresponding author
Additional information
Supplementary information is available on the website of Acta Pharmacologica Sinica.
Supplementary information
Supplementary Figure S1
Hepatoprotection of sweroside against ANIT-induced hepatotoxicity and cholestasis. (DOCX 119 kb)
Supplementary Figure S2
Serum TC, TG, LDL-C and HDL-C levels were affected by sweroside treatment. (DOCX 95 kb)
Supplementary Figure S3
Sweroside demonstrated no obvious liver toxicity by histological study and biochemical analysis. (DOCX 1968 kb)
Supplementary Figure S4
Cells viability and cells apoptosis were not affected by sweroside exposure. (DOCX 193 kb)
Rights and permissions
About this article
Cite this article
Yang, Ql., Yang, F., Gong, Jt. et al. Sweroside ameliorates α-naphthylisothiocyanate-induced cholestatic liver injury in mice by regulating bile acids and suppressing pro-inflammatory responses. Acta Pharmacol Sin 37, 1218–1228 (2016). https://doi.org/10.1038/aps.2016.86
Received:
Accepted:
Published:
Issue Date:
DOI: https://doi.org/10.1038/aps.2016.86
Keywords
This article is cited by
-
Anti-inflammatory Effects of Sweroside on LPS-Induced ALI in Mice Via Activating SIRT1
Inflammation (2021)
-
Sweroside ameliorated carbon tetrachloride (CCl4)-induced liver fibrosis through FXR-miR-29a signaling pathway
Journal of Natural Medicines (2020)
-
Protective Effects of Sweroside on IL-1β-Induced Inflammation in Rat Articular Chondrocytes Through Suppression of NF-κB and mTORC1 Signaling Pathway
Inflammation (2019)