INTRODUCTION
Among the different forms of the leishmaniasis, visceral leishmaniasis is the most dreaded one and usually fatal if not treated (Sundar and Chatterjee, Reference Sundar and Chatterjee2006; Von Stebut, Reference Von Stebut2015). To date, chemotherapy is the main weapon in our arsenal to combat this disease, which endures major issues such as resistance, variable efficacy, toxicity, parenteral administration and longer courses of administration. In the absence of an appropriate chemotherapeutic option, development of an effective vaccine has become imperative for the management of visceral leishmaniasis (VL) (Sundar et al. Reference Sundar, Pai, Kumar, Pathak-Tripathi, Gam, Ray and Kenney2001; Croft et al. Reference Croft, Sundar and Fairlamb2006; Ait-Oudhia et al. Reference Ait-Oudhia, Gazanion, Vergnes, Oury and Sereno2011). The outcome of VL is the consequence of the immune response occurred in the host wherein the systemic Leishmania infection is followed by an increase in Leishmania-specific antibodies. In addition, there is a suppression of T helper cells type 1 (Th1) cell-mediated immune response marked by downregulation of interferon gamma (IFN-γ) and interleukin-12 (IL-12) along with a significant up-regulation of T helper cells type 2 (Th2) cytokines as indicated by increase in the expression of interleukin-4 (IL-4) and interleukin-10 (IL-10) in lymphocytes (Kemp et al. Reference Kemp, Hey, Bendtzen, Kharazmi and Theander1994; Murray et al. Reference Murray, Hariprashad and Coffman1997; Coler et al. Reference Coler, Skeiky, Bernards, Greeson, Carter, Cornellison, Modabber, Campos-Neto and Reed2002; Kumari et al. Reference Kumari, Misra, Tandon, Samant, Sundar and Dube2012). In contrast, there is a typical type 1 immune response with an augmentation in the levels of IFN-γ and IL-2 as well as the infiltration of neutrophils, eosinophils and natural killer cells with an increase in IL-12 monocytes in the treated/cured cases (Karp et al. Reference Karp, el-Safi, Wynn, Satti, Kordofani, Hashim, Hag-Ali, Neva, Nutman and Sacks1993; Kemp et al. Reference Kemp, Kemp, Kharazmi, Ismail, Kurtzhals, Hviid and Theander1999; Peruhype-Magalhaes et al. Reference Peruhype-Magalhaes, Martins-Filho, Prata, Silva Lde, Rabello, Teixeira-Carvalho, Figueiredo, Guimaraes-Carvalho, Ferrari and Correa-Oliveira2005). These observations imply that any intervention, which helps to divert the Th2 type of immune response to Th1, shall play a crucial role in the management of VL. The search for parasite antigens, which could generate a desired Th1 type of cellular response should be primarily associated with the identification of novel proteins which can serve as a potential immunogen (Handman, Reference Handman2001; Coler and Reed, Reference Coler and Reed2005; Khalil et al. Reference Khalil, Musa, Modabber and El-Hassan2006).
Leishmania donovani cofactor-independent phosphoglycerate mutase (LdiPGAM) was earlier identified through proteomic study by our group in the Th1 stimulatory sub-fraction of soluble L. donovani promastigote antigen (SLD) of molecular weight ranging from 89·9 to 97·1 kDa (Gupta et al. Reference Gupta, Sisodia, Sinha, Hajela, Naik, Shasany and Dube2007; Kumari et al. Reference Kumari, Samant, Misra, Khare, Sisodia, Shasany and Dube2008b). Phosphoglycerate mutases are glycolytic enzymes, which are present in the cytoplasm of cells of different organisms, including plants, but over the last 20 years, their localization has been demonstrated in other organelles such as in cell wall of yeast, mitochondrion in Arabidopsis cells or in nucleoli of cancer cells, along with the cytoplasm (Wang et al. Reference Wang, Walling, Jauh, Gu and Lord1996; Giege et al. Reference Giege, Heazlewood, Roessner-Tunali, Millar, Fernie, Leaver and Sweetlove2003; Gizak et al. Reference Gizak, Grenda, Mamczur, Wisniewski, Sucharski, Silberring, McCubrey, Wisniewski and Rakus2015; Motshwene et al. Reference Motshwene, Brandt and Lindsey2003). PGAMs exist in two forms, which are kinetically and structurally distinguishable among the different organisms based on whether they require 2, 3-bisphos-phoglycerate as cofactor or not for their functioning. The cofactor-dependent enzyme (dPGAM) is present in all vertebrates, while cofactor independent form (iPGAM) is present in almost all nematodes, bacteria and trypanosomes with the exception to some bacteria, such as Escherichia coli and certain archaea and protozoa that contain both the iPAGM and dPAGM forms (Chander et al. Reference Chander, Setlow, Lamani and Jedrzejas1999; Chevalier et al. Reference Chevalier, Rigden, Van Roy, Opperdoes and Michels2000; Jedrzejas et al. Reference Jedrzejas, Chander, Setlow and Krishnasamy2000; Guerra et al. Reference Guerra, Vertommen, Fothergill-Gilmore, Opperdoes and Michels2004; Besteiro et al. Reference Besteiro, Barrett, Riviere and Bringaud2005; Djikeng et al. Reference Djikeng, Raverdy, Foster, Bartholomeu, Zhang, El-Sayed and Carlow2007; Foster et al. Reference Foster, Davis, Raverdy, Sibley, Raleigh, Kumar and Carlow2010). iPGAM sequences show no apparent identity with those of dPGAMs. The studies performed on the reaction mechanism of iPGAMs demonstrated that the enzyme acts in a fundamentally different way than dPGAMs (Carreras et al. Reference Carreras, Bartrons and Grisolia1980). Phosphoglycerate mutase has been molecularly characterized as iPGAM only in cutaneous form of Leishmania i.e. L. mexicana (Guerra et al. Reference Guerra, Vertommen, Fothergill-Gilmore, Opperdoes and Michels2004). As per our knowledge, iPGAM was identified by our group, for the first time, in immunogenic sub-fraction of soluble L. donovani lysate; therefore, we intend to evaluate its species-specific role. For the purpose, we first carried out molecular and biochemical characterization of iPGAM in L. donovani. Then, the ability of this recombinant protein (rLdiPGAM) to stimulate the immune responses was assessed in lymphocytes and peripheral blood mononuclear cells (PBMCs) of treated Leishmania-infected hamsters and Leishmania patients in clinical remission/endemic controls. The prophylactic potential of this recombinant protein was further assessed in hamsters against L. donovani infection.
Moreover, as this protein is unique to the parasite, there is a possibility that it can serve as a novel drug target. Therefore, development and validation of the homology three-dimensional (3D) structure of LdiPGAM was done followed by virtual screening based on docking approach. Further, Maybridge database containing 53 000 chemical compounds targeting the substrate-binding site of LdiPGAM was explored to identify its inhibitors.
MATERIALS AND METHODS
Ethics statement
Experiments on the animals (hamsters) were executed following the approval of the protocol and the guidelines of Institutional Animal Ethics Committee (IAEC) of the CDRI (reference number 154/10/Para/IAEC/2011), which is adhered to National Guideline of CPCSEA (Committee for the Purpose of Control and Supervision on Experiments on Animals) under the Ministry of Environment and Forest, Government of India.
The protocol and study with patients was approved by the Ethics committee of the Kala-azar Medical Research Centre, Muzaffarpur (Protocol # ECKAMRC/Vaccine/VL/2007–01) and a prior written consent was taken from the patients (or the parents in case if the patient was minor) before the initiation of the study. A thorough clinical examination was done by a local physician of all the human subjects in the study for Leishmanial and other possible infections.
Animals
Laboratory-bred male golden hamsters (Mesocricetus auratus, 45–50 g) from the Institute's animal house facility were used as hosts for the experiments. They were housed in a climatically controlled room and fed with standard rodent food pellet (Lipton India, Mumbai, India) and water ad libitum. The experimental animals were monitored daily till the termination of the study. In order to reduce the suffering level in experimental animals, hamsters were autopsied at the endpoint by deep anaesthesia with over dosages of sodium thiopentone (50 mg kg−1).
Parasites and macrophage cell line
Leishmania donovani clinical strain (2001), which is SAG-sensitive isolate, was procured from a Kala-azar patient from Banaras Hindu University, Varanasi Uttar Pradesh, India, in the year 2000. The promastigotes were cultured in complete Roswell Park Memorial Institute-1640 (RPMI-1640) medium containing 10% heat-inactivated fetal bovine serum (Sigma, USA), 100 U mL−1 penicillin (Sigma) and 100 mg mL−1 streptomycin (Sigma) at 26 °C. The maintenance of parasite was also carried out in hamsters through serial passages (i.e., from amastigote to amastigote) (Dube et al. Reference Dube, Singh and Sundar2005).
Mouse macrophage cell line J774A.1 obtained from the institute's Tissue culture facility was cultured in RPMI-1640 through sub-culture in 25 cm2 flasks at kept at 37 °C and 5% CO2.
Soluble L. donovani promastigote antigen
SLD was prepared following the protocol described by Gupta et al. (Reference Gupta, Sisodia, Sinha, Hajela, Naik, Shasany and Dube2007). The estimation of protein content of the supernatant was done by the Bradford method and the antigen was stored at −70 °C till further use.
Cloning, expression and purification of rLdiPGAM
The designing of gene-specific primers was carried out according to the gene sequence of L. mexicana iPGAM available in the database. PCR amplification using the L. donovani genomic DNA as template was performed with the oligonucleotides: 5′-GGATCCATGTCAGCACTTCTCTTGAAG-3′ as a forward primer and 5′-GAATTCCTTCTCGACGTAGATCAGGCTAG-3′ as reverse primer containing BamHI and EcoRI restriction sites (underlined) respectively. Using 2× PCR Master mix (Fermentas, USA) LdiPGAM gene was amplified, and the product was inserted in-frame with a 6× His tag into pET-28a vector (Novagen, USA) at BamHI/EcoRI site, and it was confirmed by sequencing. E. coli BL21-Codon plus (DE3) strain competent cells were used to express L. donovani rLdiPGAM and following standard procedure the recombinant protein with N-terminal His-tag was purified employing Ni-NTA agarose column beads (Qiagen, Germany) in lysis buffer (50 mm Tris, 500 mm NaCl and 10 mm imidazole; pH 8·0). The eluted protein was resolved on 12% sodium dodecyl sulphate polyacrylamide gel electrophoresis (SDS–PAGE) and stained with the Coomassie Brilliant Blue stain (Sigma). The protein was quantified by the Bradford method using bovine serum albumin (BSA) as standard. The protein was also subjected to Limulus amoebocyte lysate test (QCL-1000, Lonza, USA) to detect the presence of endotoxin content.
Polyclonal antibodies production against rLdiPGAM and Western Blotting
The antibodies against purified rLdiPGAM protein were developed in Balb/c mice. Mice were first immunized with 25 µg of recombinant protein in Freund's complete adjuvant. The mice were given three booster doses of 10 µg protein, each in incomplete Freund's adjuvant, at 2-weeks interval and the serum was collected by sacrificing the mice on 8th day after the last immunization. The titre of antibody was verified by enzyme-linked immunosorbent assay (ELISA). For western blotting, purified protein, Leishmania promastigote whole cell lysate, and SLD were resolved on 12% SDS–PAGE and transferred onto nitrocellulose membrane electrophoretically employing Hoefer Semiphor semi-dry blot apparatus (Hoefer, USA). The membrane, after blocking in 5% skimmed milk overnight, was incubated with anti rLdiPGAM antibodies (1:1000 dilution) for 2 h at room temperature (RT). After washing the membrane three times with phosphate buffer saline (PBS) + 0·5% Tween 20 (PBS-T), it was incubated with goat anti-mouse immunoglobulin G (IgG) horseradish peroxidase-conjugated antibody at a dilution of 1: 10 000 for 1 h at RT and then the blot was developed using the Enhanced chemiluminescence kit (Invitrogen, USA).
Size exclusion chromatography
Approximately 500 µL (~200 µg) of purified recombinant protein was loaded onto a Sephdex-75 10/300 column (GL column), which was pre-equilibrated with buffer (50 mm Tris–HCl, pH 7·2, 300 mm NaCl) using a manual injector. Chromatography was performed on an AKTA purifier system (GE Healthcare, USA) at a flow rate of 0·3 mL min−1 at 25 °C and the absorbance was monitored at 280 nm. The column was calibrated with standard molecular weight markers.
Immunolocalization
The promastigotes, washed with 1× PBS, were stained with Mito Tracker dye (500 nm in PBS, Invitrogen, USA) for 20 min. The cells were then washed with PBS, fixed in paraformaldehyde and allowed to adhere to poly-(L-lysine)-coated coverslips. Thereafter, the permeabilization of the parasites was done using 0·2% (v/v) Triton X-100 and they were washed again with PBS. The fixed cells were incubated for 30 min in PBS containing 3% BSA and washed again with PBS solution. Following this the parasites were incubated with mouse anti-LdiPGAM primary antibody at a dilution of 1: 10 000 for 1 h, washed with PBS and were incubated again for 1 h with FITC-conjugated goat anti-mouse IgG. The coverslips, after washing with PBS were treated with PBS containing Propidium Iodide (2 µg mL−1). Then, these were mounted on fresh slides using 10 µL of Fluorescent mounting media (Merck) and finally observed under confocal laser-scanning microscope (Olympus Fluoview FV1000, Japan).The negative control samples, which were devoid of primary antibodies were processed in parallel to negate background fluorescence.
Leishmania cell synchronization and analysis of LdiPGAM expression in different stages of cell cycle
Leishmania donovani cultures were synchronized by treating log phase cultures (2–3 × 107 cells mL−1) with 5 mm hydroxyurea (HU) for 12 h. Afterward, parasites were harvested, washed twice with PBS and released into a drug-free RPMI-1640 medium, as described previously (Minocha et al. Reference Minocha, Kumar, Rajanala and Saha2011). For flow cytometric analysis 1–2 × 107 synchronized promastigotes were harvested at different time intervals after HU treatment, washed with PBS, fixed in 30% PBS + 70% methanol overnight at 4 °C, collected by centrifugation, washed with PBS, and stained in PBS containing propidium iodide (10 µg mL−1) as well as RNase (10 µg mL−1) at 37 °C for 30 min. Flow cytometric analysis was carried out using a BD FACS Calibur™ flow cytometer and the CellQuest Pro software (BD Biosciences, USA). G1, S and G2/M phases are indicated on the histograms by the gates M1, M2 and M3, respectively, which were derived from the data using the CellQuest Pro software. LdiPGAM expression in synchronized cells was analysed at different times by western blot analysis (10% PAGE) of whole cell extracts of 2 × 107 using anti-LdiPGAM antibody (1:1000 dilution). Quantitative assessment was carried out with Image-J software (NIH IMAGE) and the expression level was normalized to Grp78.
Kinetic analysis of rLdiPGAM
Enzyme assays
The enzymatic activity of rLdiPGAM was measured by following either the increase of UV absorbance at 240 nm due to phosphoenolpyruvate (PEP) production (molar extinction coefficient 1310 m−1 cm−1) or the decrease in UV absorbance at 340 nm due to nicotinamide adeninie dinucleotide hydrate (NADH) oxidation (molar extinction coefficient 6250 m−1 cm−1) (1) using a spectrophotometer (SpectraMax, USA).
PEP production, forward reaction
The reaction was followed upon the addition of 1·5 mm 3-phosphoglycerate (3PGA) into a 1 mL quartz cuvette containing 50 mm Tris–HCl (pH 7·6), 0·55 U of rabbit muscle enolase (ENO), 1 mm MgCl2, 0·1 mm CoCl2 and 50 mm KCl. One activity unit (U) is defined as the conversion of 1 µmol substrate min−1 under standard conditions. Auxiliary enzymes used were obtained from Sigma-Aldrich, USA.
NADH oxidation, reverse reaction
The conversion of 2-phosphoglycerate (2PGA) to 3PGA was coupled to NADH oxidation by glyceraldehyde-3-phosphate dehydrogenase (GAPDH) via 3-phosphoglycerate kinase (PGK). The assay was carried out at 25 °C in a 1 mL reaction mixture containing 0·1 m Tris–HCl pH 7·6, 5 mm MgCl2, 1 mm dithiothreitol, 1 mm ATP, 0·56 mm NADH, 0·01 mm CoCl2, 0·8 mm 2PGA and GAPDH and PGK both at 6 U mL−1.
Kinetic constants were determined using freshly purified rLdiPGAM. As NaCl, CoCl2 and imidazole are required for the stability of the recombinant protein (Guerra et al. Reference Guerra, Vertommen, Fothergill-Gilmore, Opperdoes and Michels2004), the recombinant enzyme was pre-dialysed against 50 mm Tris–HCl (pH 7·6), 100 mm NaCl, 0·1 mm CoCl2 and 50 mm imidazole (so as to get its optimum activity) and protein concentration was determined. For K m calculation of forward reaction 11 assays were performed spanning a range of different concentrations of 3PGA from 0·05 to 3·0 mm. For K m calculation of the reverse reaction, eight assays were performed spanning different concentrations of 2PGA ranging from 0·05 to 0·4 mm. Optimum curve fitting of data to the Lineweaver–Burk equation was calculated.
The pH optima of LdiPGAM were determined using forward reaction in Tris–HCl buffer at various pH ranging between 3·0 and 9·0.
Homology modelling and molecular docking studies of LdiPGAM
The sequence of LdiPGAM consisting of 553 amino acid residues was retrieved from the Uniprot database (accession code: D7R810). This sequence was used as a query for NCBI Blastp to search a suitable template. The LdiPGAM homology modelling was done using the crystal structure of Leishmania mexicana cofactor-independent phosphoglycerate mutase (LmiPGAM) (PDB ID: 3IGZ) (Nowicki et al. Reference Nowicki, Kuaprasert, McNae, Morgan, Harding, Michels, Fothergill-Gilmore and Walkinshaw2009) as the template. ClustalW program (Chenna et al. Reference Chenna, Sugawara, Koike, Lopez, Gibson, Higgins and Thompson2003) was used to perform sequence alignment. For the homology modelling MODELLER9.10 (Sali and Blundell, Reference Sali and Blundell1993) software was used, that generated 20 models for LdiPGAM and rated according to GA341 and DOPE scoring functions available with it. ‘Loopmodel’ class present in MODELLER was used for the refinement of loop regions. The best quality predicted 3D structure was selected using PROCHECK, which was used for further study (Laskowski et al. Reference Laskowski, MacArthur, Moss and Thornton1993). All the analysis and visualization of the structure files were done using chimera (Pettersen et al. Reference Pettersen, Goddard, Huang, Couch, Greenblatt, Meng and Ferrin2004).
To validate the active site of modelled protein and to check the reliability of our docking program, first 3PGA was docked into modelled LdiPGAM structure using the program DOCK6.5 (Pettersen et al. Reference Pettersen, Goddard, Huang, Couch, Greenblatt, Meng and Ferrin2004). Also for the in silico screening of over 53 000 compounds of Maybridge screening database, this DOCK6.5 program was utilized. The Maybridge database was prepared by converting all the structures present in it into 3D mol2 format from the 2D sdf format using CONCORD module of SYBYL7.1 program [SYBYL Molecular Modeling System Version 7.1 (2005), Tripos Inc., St. Louis, MO]. For docking studies, protein preparation and docking procedures were performed according to the standard protocol implemented in DOCK6.5. On the receptor surface, receptor spheres were placed using the sphgen program within the DOCK package. By using grid program of DOCK package, receptor electrostatic and VDW grid points were calculated. Each Maybridge screening compound was docked as a flexible body in different orientation and energy minimization was enabled. The ligand–receptor interaction energy predicted by DOCK6 is based on the grid method and it is calculated as the sum of electrostatic and van der Waals components. The conformation of each of the ligand with most favourable interaction energy was chosen for further study.
Enzyme inhibition assay
The top five scoring compounds from Maybridge library were selected for enzyme inhibition assay.
Forward reaction, in which PEP production is measured, was used for the enzyme inhibition assay. All the test compounds were dissolved in dimethyl sulfoxide (DMSO) at 0·5 m concentration. Dilution with triple distilled water was carried out to make their final concentrations of 10, 25, 50 and 100 µ m for each test compound. The final concentrations of DMSO used were 0·00002, 0·00005, 0·0001 and 0·0002% (in 10, 25, 50 and 100 µ m of the test compounds, respectively). The inhibition assay was carried out by preincubating 20 µg of LdiPGAM with 10, 25, 50 and 100 µ m concentrations of each compound (inhibitors) for 1 h at 37 °C. The reaction started with the addition of the enzyme reaction buffer (50 mm Tris–HCl, pH 7·6) and other substances as described above into the LdiPGAM–inhibitor mixture to reach the final volume of 1 mL. The blank wells contain all components of the reaction mixture including DMSO, but not any test compound to check any detergent effect. Furthermore, in order to ascertain that the inhibitory effect of the compounds were specific for LdiPGAM and not for the auxilay enzyme ENO, activity of the ENO enzyme was assessed in the presence of different concentrations of the inhibitors by measuring PEP production using 2PGA as the substrate. The assay was performed at 25 °C in a 1·0 mL reaction mixture containing 50 mm Tris–HCl (pH 7·5), 1 mm MgCl2, 50 mm KCl and 1 mm 2PGA. All the assays were performed in three replicates, and their means were calculated.
Immunogenecity of rLdiPGAM in lymphocytes/PBMCs of treated Leishmania infected hamsters and Leishmania patients in clinical remission
Treatment of L. donovani-infected hamsters and isolation of lymphocytes
The hamsters (~20) were intracardially infected with 107 L. donovani amastigotes and assessed for parasitic burden by splenic biopsy 1 month later as mentioned earlier (Singh et al. Reference Singh, Kumar, Gupta, Dube and Lakshmi2008). Those animals which harbour 25–30 amastigotes/100 macrophage cell nuclei were then subjected to the antileishmanial therapy with Miltefosine (Zentaris, Germany) continually for 5 days at a dose schedule of 40 mg kg−1 body weight. On day 30 post treatment the animals were reexamined for the clearance of parasites by performing splenic biopsy.
For lymphoproliferative assay and NO production the lymph node cell suspension obtained from mesenteric lymph nodes of normal (uninfected), L.donovani infected (untreated) as well as treated hamsters as per the procedure described by Garg et al. Reference Garg, Gupta, Tripathi, Naik, Sundar and Dube2005). The cells were counted and a suspension of 106 cells mL−1 was made in complete RPMI 1640 medium.
Human samples and isolation of PBMCs
Human samples were taken as per study groups described below:
(1) Seven Leishmania patients in clinical remission (four males and three females, age ranging from 5 to 40 years) from hyperendemic areas of Bihar. The Leishmania patients, treated with a complete course of amphotericin B were checked for the parasite clearance in splenic aspirates. Blood samples were collected from these patients 2 months to 1 year post treatment.
(2) Seven endemic controls (four males and three females; age range 15–45 years) belonging to the family of infected and/or Leishmania patients in clinical remission (household contacts) that neither showed clinical symptoms nor received any treatment for Kala-azar.
(3) Seven Leishmania-infected patients (three males and four females, age range 5– 40 years) showing clinical symptoms of Kala-azar.
(4) Seven normal healthy donors (three males and four females, age range 25–30 years) from non-endemic areas, without any history of leishmaniasis, served as negative control.
The study was approved by the ethics committee of the Kala-azar Medical Research Centre, Muzaffarpur, India (protocol number ECKAMRC/Vaccine/VL/2007-01).
Heparinized venous blood (10 mL each) was collected from all of the study subjects, and PBMCs were isolated from blood by Ficoll–Hypaque density gradient centrifugation (Histopaque 1077; Sigma-Aldrich) as described by Garg et al. (Reference Garg, Gupta, Tripathi, Naik, Sundar and Dube2005). After determining the cell viability by trypan blue staining method, the 106 cells mL−1 was resuspended in a complete RPMI 1640 medium and was used for following immunological assays:
Assessment of lymphocyte proliferative responses
The assay for lymphoproliferative responses was done following the method of Garg et al. (Reference Garg, Gupta, Tripathi, Naik, Sundar and Dube2005) with slight modifications, wherein, a non-radioactive XTT [2,3-bis-(2-methoxy-4-nitro-5-sulfophenyl)-2H-tetrazolium-5-carboxanilide] reagent (Roche Diagnostics, USA) was used instead of radioactive [3H] thymidine. Lymph node cell suspension from hamsters or PBMCs from human blood samples (1 × 106 cells mL−1) was cultured in 96-well flat-bottom tissue culture plates (Nunc). The cells were stimulated with 100 µL of mitogens [phytohaemaglutinin (PHA; Sigma-Aldrich, USA) for human PBMCs and concanavalin A (Sigma-Aldrich, USA) for hamster's lymphocytes] as well as antigens (rLdiPGAM and SLD) in triplicate at a concentration of 10 µg mL−1 for each of PHA, Con A, rLdiPGAM and SLD. The cultures were kept at 37 °C in a CO2 incubator with 5% CO2 for 3 and 5 days respectively for mitogens and antigens. After that, 50 µL of XTT (Roche Diagnostics, USA) was added to each well and the cultures were incubated further for 4 h. Finally, the absorbance was measured at 480 nm with 650 nm as reference wavelength.
Induction of NO production by in hamster lymphocytes/PBMCs from patients
The lymph node cells from hamsters (or PBMCs from patients) were suspended in a culture medium, plated at 105 cells well−1, and stimulated for 3 days in case of mitogen (LPS) and 5 days in case of antigens (rLdiPGAM, SLD) at 10 µg mL−1. The presence of NO was assessed using Griess reagent (Sigma-Aldrich, USA) in the culture supernatants of macrophage cell lines (J774 A.1) after the exposure with supernatant of stimulated lymphocytes. The supernatants (100 µL) collected from macrophage cultures 24 h after incubation was mixed with an equal volume of Griess reagent and left for 10 min at RT. The absorbance of the reaction was measured at 540 nm in an ELISA reader (Ding et al. Reference Ding, Nathan and Stuehr1988). The nitrite concentration in the macrophages’ culture supernatant samples was extrapolated from the standard curve plotted with sodium nitrite.
Assessment of cytokine levels-IFN-γ/interleukin-12 (IL-12P40)/IL-10 in lymphocytes of cured/endemic patients
Culture of PBMCs (1 × 106 cells mL−1) from human patients was set up in 96-well culture plates, and rLdiPGAM was added at a concentration of 10 mg mL−1 in triplicate wells. The level of IFN-γ, interleukin-12 (IL-12p40) and IL-10 was estimated by ELISA kit (OptEIA set; BD Pharmingen, USA) after 5 days of incubation with antigens using supernatants. The results were expressed as pictogram (pg) of cytokine mL−1, based on the standard curves of the respective cytokine provided in the kit. The lower detection limits for various cytokines were as follows: 4·7 pg mL−1 for IFN-γ, 7·8 pg mL−1 for IL-12p40 and 7·0 pg mL−1 for IL-10.
Determination of in vivo immunomodulation by LdiPGAM in naïve hamster
Two groups of animals each containing five hamsters were immunized intradermally (i.d.) on the back with rLdiPGAM (50 µg) and PBS, respectively. After 15 days, a booster dose of half of the amount of antigen and PBS each was given to the hamsters of both the experimental groups. Thirty days later, the animals were sacrificed, and their spleens were isolated to extract RNA to assess the level of cytokines by quantitative real-time polymerase chain reaction (qRT–PCR).
Quantification of mRNA cytokines and inducible NOS in hamsters by qRT–PCR
qRT–PCR was performed to assess the expression of mRNAs for various cytokines and inducible NO synthase (iNOS) in splenic cells. Splenic tissues were taken from each of the three randomly chosen animals. Total RNA was isolated using TRI reagent (Sigma-Aldrich, USA) and quantified by using Gene-quant (Bio-Rad, USA). One microgram of total RNA was used for the synthesis of cDNA using a first-strand cDNA synthesis kit (Fermentas, USA). For real-time PCR, primers were designed using Beacon Designer software (Bio-Rad, USA) on the basis of cytokines and iNOS mRNA sequences available on PubMed (Melby et al. Reference Melby, Tryon, Chandrasekar and Freeman1998a) (Table 1). qRT–PCR was conducted as per the protocol described earlier (Samant et al. Reference Samant, Gupta, Kumari, Misra, Khare, Kushawaha, Sahasrabuddhe and Dube2009) by using SYBR Green PCR master mix (Bio-Rad, USA). Results were expressed as the fold expression change of mRNA level between control and experimental groups.
Table 1. Sequences of forward and reverse primers of hamster cytokines used for quantitative real time RT–PCR

Vaccination with rLdiPGAM in hamsters
For immunization, a total of 60 Syrian golden hamsters (40–45 g) were divided into four groups, each containing 15 animals, wherein groups 1 and 2 served as normal control as well as infected controls, respectively, while groups 3 and 4 as the Bacillus Calmette–Guérin (BCG) as well as vaccinated groups. The hamsters of group 4 were injected i.d. with 100 µg of rLdiPGAM in 100 µL PBS along with equal volume of BCG. Fourteen days later, a booster dose of 50 µg of rLdiPGAM + BCG was given i.d. to group 4. Day 21 after vaccination, each animal of groups 2, 3 and 4 was challenged intracardially with 1 × 106 amastigotes of the clinical strain of L. donovani. On days 45 and 60 post challenge (p.c.), three to five hamsters per group were necropsied for the assessment of parasitological and immunological (Lyphoproliferation, NO, cytokines by RT–PCR and antibody level by ELISA) progression of VL (Gupta et al. Reference Gupta, Kushawaha, Tripathi, Sundar and Dube2012). The assessment of parasitic burden was made by counting the number of amastigotes per 1000 cell nuclei in the impression smears of different organs, namely spleen, liver and bone marrow (femur bone) of experimental animals. The percentage estimation of inhibition of parasite multiplication was done in comparison with the unvaccinated control using the following formula:

Simultaneously, for evaluation of cellular and antibody responses at these time points, peritoneal exudate cells, mesentric lymph nodes and blood were also collected to obtain cells and sera.
In addition, level of antileishmanial IgG antibodies and its isotypes was measured in sera samples of different experimental groups of hamsters following the slightly modified protocol of Samant et al. (Reference Samant, Gupta, Kumari, Misra, Khare, Kushawaha, Sahasrabuddhe and Dube2009). Briefly, overnight coating of 96-well ELISA plates (Nunc, USA) with rLdiPGAM (0·2 µg/100 µL well−1) at 4 °C and blocking with 1·5% BSA at RT for 1 h was carried out. Sera were used as a primary antibody and added at a diluted ratio of 1:100 for 2 h at RT. Secondary antibodies in the dilution of 1/1000 form of Biotin-conjugated mouse anti-Syrian hamster IgG, IgG1 and biotinylated anti-Syrian hamster IgG2 (BD Pharmingen, USA) were added for 1 h at RT respectively and were incubated further for 1 h with peroxidase conjugated streptavidin at 1/1000 (BD Pharmingen, USA). Finally, after putting in the substrate O-phenylenediamine dihydrochloride (Sigma-Aldrich, USA), reading of the plate was taken at 492 nm.
Post-challenge survival
Six animals from each group were observed for their physical conditions and were given proper care, until their survival period. Mean survival period was calculated from the recorded survival data of individual hamster.
Statistical analysis
Two sets of experiments were performed and the results were expressed as mean ± s.d. The analysis of the results was done by Student's t test and one-way ANOVA test followed by Dunnets or Tukey's post hoc test, whichever was appropriate in each case, using the GraphPad Prism software program (GraphPad). The value of P < 0·05 was considered as significant.
RESULTS
rLdiPGAM was cloned, expressed, purified and antibody against the protein was raised
The amplification and cloning of LdiPGAM gene was successfully carried out in pTZ57R/T (T/A) cloning vector (Fig. 1A and B). The gene was transformed into competent E. coli DH5α cells and the positive ones were sequenced from Chromous Biotech Pvt. Ltd. The sequenced gene was submitted to the National Centre for Biotechnology Information (Accession no. HM044147). A sequence analysis using the NCBI database (www.ncbi.nlm.nih.gov) revealed that LdiPGAM has close similarity with L. infantum and other species of Leishmania (Supplementary Table S1). The gene was further sub-cloned into pET28a vector and the recombinant plasmid was characterized with double enzymatic digestion (Fig. 1C) and checked for overexpression. 1659 bp amplicon of LdiPGAM gene encoded 553 amino acids with a predicted molecular mass of ~60 kDa. Comparisons of both non-induced and induced culture in SDS–PAGE revealed that the protein was expressed successfully and the induced protein bands corresponded to its predicted size (Fig. 1D). Purification of His-tagged rLdiPGAM by metal affinity chromatography yielded of pure protein (Fig. 1D). The LPS content of the purified recombinant proteins was estimated to be below 5 endotoxin units (EU)/mg of the recombinant protein as measured by Limulus amoebocyte lysate test. Further, titre of the antibody produced against the recombinant protein was in the ratio of 1: 25 600 as determined by ELISA. Western Blotting using the antiserum to rLdiPGAM, detected single band at ~60 kDa in the soluble promastigote lysate, thus exhibiting its specificity (Fig. 1E). By size exclusion chromatography of this purified rLDiPGAM, a single elution peak at ~67 kDa was observed on Sephadex G-75 column chromatography (Fig. 1F), which revealed the monomeric nature of the protein thus confirming the presence of cofactor-independent form of the enzyme in L. donovani parasite.

Fig. 1. Cloning overexpression, purification and raising of antibody against rLdiPGAM (A) Specific PCR of LdiPGAM; lane 1: 1 kb ladder, lanes 2–3: amplified PCR product at 1659 bp. (B) Clone confirmation in pTZ57R/T; lane 1: 1 kb ladder, lane 2: BamHI/EcoRI digested pTZ57R/T + LdiPGAM, lane 3: Undigested plasmid (C) Clone confirmation in pET28a, Lane 1: 1 kb ladder, lane 2: BamHI/EcoRI HI digested pET28a + LdiPGAM, lane 3: Undigested plasmid (D) Expression and purification of rLdiPGAM in E. coli cells; Whole Cell Lysate (WCL) of transformed E. coli separated on 10% acrylamide gel and stained with Coomassie Brilliant Blue. Lane 1: WCL before IPTG induction, lane 2: WCL after IPTG (1·0 mm) induction at 37 °C; lanes 3–5 wash fractions and lanes 6–8 eluted protein and lane 9: Molecular weight marker. (E) Western Blot analysis using anti-rLdiPGAM Ab in uninduced WCL, induced WCL and Leishmania WCL and soluble Leishmania antigen (SLD). Lane 1: Molecular weight marker; lane 2: Uninduced E. coli WCL; lane 3: induced E. coli WCL; lane 4: purified rLdiPGAM; lane 5: Leishmania WCL; and lane 6: SLD. (F) Size exclusion chromatographic profile of rLdiPGAM carried using a Sephdex-75 10/300 column (GL column) pre-equilibrated with buffer (50 mm Tris–HCl, pH 7·2, 300 mm NaCl). Single elution peak of rLdiPGAM was observed in chromatographic profile.
Immunolocalization
To find out the subcellular location of the rLdiPGAM protein, the immunolocalization studies was carried out, which revealed the presence of the enzyme in whole cytoplasm of the cell (Fig. 2A)
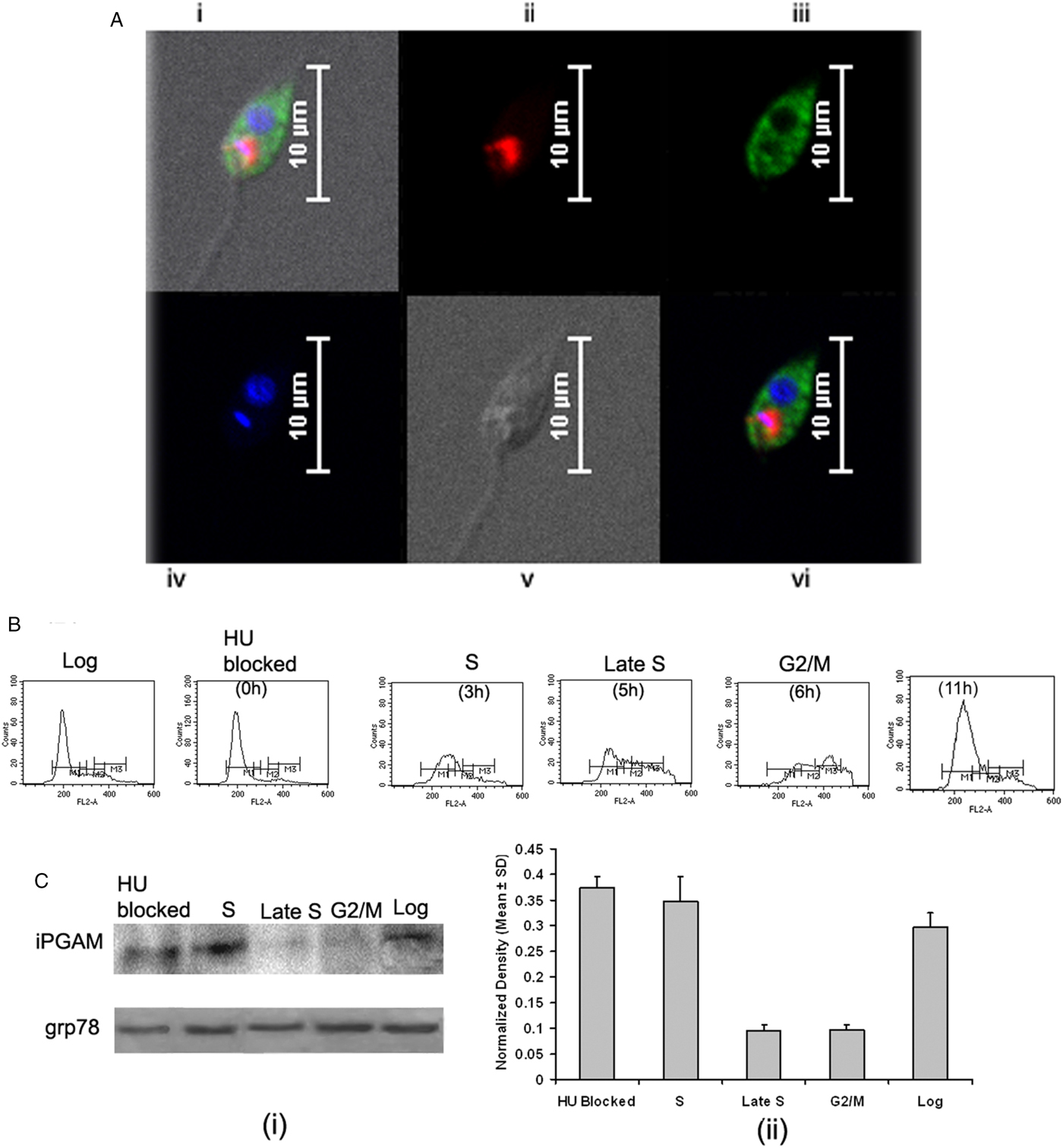
Fig. 2. Intracellular localization of LdiPGAM and assessment of its expression in different stages of Leishmania cell cycle. (A) Immunofluorescence analysis of the intracellular distribution of LdiPGAM in L. donovani promastigotes: (i) merged image of all fields; (ii) kinetoplast labelling with mitotracker dye; (iii) immunolocalization image of LdiPGAM; (iv) cell nucleus and kinetoplastic nucleus labelled with DAPI; (v) differential interference contrast image; (vi) merged image of all fluorescence images. (B) Flow cytometry analysis of hydroxyurea (HU)-treated synchronized cells harvested at different time intervals. (C) (i) Western Blot analysis (10% PAGE) of whole-cell extracts (2 × 107) cell equivalents) at different phases of cell cycle using anti-LdiPGAM antibody (1:1000 dilution); (ii) Bar chart represents mean and s.d. expression of LdiPGAM relative to grp78 in three independent experiments.
Differential expression of LdiPGAM in different phases of cell cycle
LdiPGAM expression was measured in different phases of cell cycle by synchronizing the cells. It was evident from flow cytometry analysis that HU treatment of the parasites was arresting Leishmania cell cycle at the G1/S phase boundary. At 12 h post HU treatment, most of the cells were found to be arrested in the G1 phase. When HU was removed, the parasites proceeded through the cell cycle. The cells returned to the log phase after 11 h as observed at regular interval of time (Fig. 2B). The expression of LdiPGAM was assessed in different phases of cell cycle by Western Blot analysis. Maximum expression of the protein was observed in G1 and S phases, which was decreased by ~4-fold in late S and G2/M phases [Fig. 2C(i) and (ii)].
Kinetic analysis of rLdiPGAM
The recombinant protein thus obtained has been subjected to a preliminary kinetic analysis. Under the conditions described above, the following values for the Michaelis constants were measured: for the forward reaction, the K m = 0·302 ± 0·017 mm for 3PGA substrate; for the reverse reaction, the K m = 0·143 ± 0·012 mm for 2PGA. These K m values of the recombinant were observed to be within the same range as reported for iPGAM from other organisms (Britton et al. Reference Britton, Carreras and Grisolia1971; Fothergill-Gilmore and Watson, Reference Fothergill-Gilmore and Watson1989; Guerra et al. Reference Guerra, Vertommen, Fothergill-Gilmore, Opperdoes and Michels2004; Erfe et al. Reference Erfe, David, Huang, Lu, Maretti-Mira, Haskell, Bruhn, Yeaman and Craft2012). Specific activity of the enzyme for forward and reverse reaction was calculated as 5·26 and 0·675 µm min−1 mg−1 of protein respectively. Optimum pH for the enzyme activity was found to be in the range of 7·5–8.
Homology modelling of LdiPGAM and molecular docking followed by enzyme inhibition assay
LdiPGAM has 92% of sequence identity with crystal structure of LmiPGAM, which was selected as its template for homology modelling (Fig. S1A), and the superimposition of the modelled complex with template shows a RMSD of 0·149 Å (Fig. S1B). All models generated in MODELLER9.10 were analysed by SAVES server. Ramachandran plot generated by PROCHECK for the final selected model showed 93·2% residues in the most favoured region and 6·1, 0·6 and 0·0% residues lie in additional allowed region, generously allowed and disallowed region, respectively (Fig. S1C). The structure of protein made of 553 amino acid residues and consist of two domains namely phosphatase and transferase, which are present in close proximity with active site. The phosphatase domain starts from 84 residues located at N-terminal and the transferase domain (Ala92 to Asn326) is connected with phosphatase domain through first inter domain loop (Gly85 to Gly91). However, the transferase domain is connects back with phosphatase domain with second inter domain loop, which runs from Phe327 to Arg336. The active site interacts with the substrate as well as product and also the metal ion and it comprises 16 residues. The data from previous x-ray and crystallization studies suggest that iPGAM exists in equilibrium between two extreme conformations in which the two-domain monomer may be closed with an inaccessible active site, or open with an accessible active site. In the closed form the enzyme (as in Fig. S1B) catalyses 2/3PGA isomerization resulting in product release, and product release causes opening of the enzyme and return to the open conformation (Blackburn et al. Reference Blackburn, Fuad, Morgan, Nowicki, Wear, Michels, Fothergill-Gilmore and Walkinshaw2014).
LdiPGAM is an important enzyme of glycolysis pathway, so inhibition of LdiPGAM has become an attractive target for the design of anti-leishmanial inhibitors. An additional advantage in choosing this as target is that LdiPGAM and human PGAM are not homologous and thus share no common features. On this basis, LdiPGAM was selected as the target receptor for docking of Maybridge screening database. The modelled structure of LdiPGAM in complex with metal ions was used for docking. Zinc parameters were used for docking. Modelled protein and template protein have 92% of sequence identity; consequently, they share identical active site and metal-binding site. The substrate 3PGA was extracted from the x-ray crystal structure of template protein, and then docked in the active site of LdiPGAM. The conformation of the docked substrate in modelled protein complex was found similar to that of template protein and the substrate occupied the same active site as in template.
In the above-mentioned active site, approximately 53 000 small compounds of Maybridge screening database were docked in different orientations and ranked by energy score. The top five scoring compounds were selected for experimental testing and these compounds were found to display binding mode similar to that of the substrate within the active site of LdiPGAM, which was analysed visually. Our experimental results also show that two out of five selected compounds are inhibiting enzymatic activity significantly. Enzyme assays with forward reaction was performed to confirm the inhibitory role of these compounds on rLdiPGAM. TL00638 (1-methyl-1H-imidazole-4,5-dicarboxylic acid) exhibited maximum inhibitory activity followed by SPB00184 (2-hydroxyiminoindane-1,3-dione) as shown in Fig. S2A. IC50 for TL00638 and SPB00184 were observed to be 37·3 ± 0·9 and 60·9 ± 3·5 µm (mean ± s.d.), respectively. Moreover, these compounds did not affect the activity of the auxiliary enzyme ENO (data not shown), which indicates that the compounds are specific inhibitors of LdiPGAM. In order to reveal the mechanism of inhibition by these two compounds, kinetic analysis was performed, which showed the competitive inhibition of enzyme activity by these compounds (Fig. S2B). To understand the mechanism for the inhibition of LdiPGAM by two selected compounds, we analysed the docking modes of substrate as well as selected compounds in the active site of LdiPGAM. Both substrates and inhibitors fit well in the active site pocket with several hydrogen bond interactions with the active site residues. The binding conformation of these compounds is shown in Fig. S3. The substrate 3PGA of LdiPGAM, anchored to the cavity by the hydrogen-bonding interactions with Ser75, His136, Arg167, Arg202, Arg209, Arg282, Arg285 and His360. The nitrogen atom of imidazole group of compound TL00638 forms a hydrogen bond with side-chain N–H of Arg166 and Arg285. The oxygen atoms of the dicarboxylic acid group of this compound forms hydrogen bonds with Ser75, His136, Asp167, Arg202 and Arg282. A similar binding mode is also observed for the binding of compound SPB00184. Here cyclopentane moiety is basically involved in hydrogen bond interaction. The nitrogen of oxime group forms two hydrogen bonds with side-chain nitrogen of Arg209. The carbonyl oxygens attached with cyclopentane ring forms hydrogen bond with Ser75, Arg166, Arg282 and Arg285.
rLdiPGAM induced lymphoproliferation and NO production in treated Leishmania-infected hamsters
Assessment of cellular responses of lymphocytes from treated Leishmania-infected hamsters with Con A as well as rLdiPGAM was done by XTT (Roche Diagnostics, USA) and was compared with that of SLD-stimulated group serving as control. The lymphocytes from normal as well as treated Leishmania-infected hamsters have revealed significantly higher proliferative responses when stimulated with Con A (P < 0·001) as well as rLdiPGAM (P < 0·05) in comparison with the SLD-stimulated ones (Fig. 3A); whereas in infected hamsters only Con A (P < 0·05) induced significant proliferation than SLD.

Fig. 3. Lymphoproliferative and NO response of rLdiPGAM in cured hamsters. (A). Lymphoproliferative response of mononuclear cells of lymph nodes from normal, and L. donovani infected and treated hamsters against recombinant protein/SLD. (B) NO production by peritoneal macrophages, in response to the recombinant protein/SLD stimulation. Results are expressed as means and s.d. for three to four hamsters per group done in triplicate and are representative of two independent experiments with similar results. The analysis of the results was done by the one-way ANOVA test followed by Tukey's post test. Significance values indicate the difference between the SLD and the recombinant proteins stimulation P < 0·05(*), P < 0·01(**) and P < 0·001(***).
The presence of NO was assessed in the culture supernatants of mouse J774A.1 macrophages after their exposure to supernatants of stimulated lymphocytes. There was 1·6-fold higher NO production in rLdiPGAM-stimulated cells as compared with SLD stimulated one (P < 0·05) (Fig. 3B).
rLdiPGAM induces PBMCs from Leishmania patients in clinical remission/endemic individuals to proliferate and to generate a predominant Thl cytokine responses
PBMCs from the patients in clinical remission, endemic and non-endemic controls and L. donovani-infected donors were used to further analyse their cellular and cytokine responses. Proliferation and cytokine responses of PBMCs from patients with active VL/cured/endemic against rLdiPGAM and SLD were compared, while PHA stimulation served as positive control. Since PHA is a mitogen, so all the groups stimulated with PHA showed significantly higher OD than SLD and rLdiPGAM (P < 0·001 compared with SLD and P < 0·01 with rLdiPGAM). Cells from the patients in clinical remission show better proliferative responses against rLdiPGAM with mean OD value of 1·087462, which is higher than SLD (0·708671) (P < 0·01) (Fig. 4A). Similarly, rLdiPGAM induces significant NO production in PBMCs of patients in clinical remission and endemic controls (P < 0·05) (Fig. 4B).The results indicate towards the recognition of rLdiPGAM as a potent T-cell antigen by majority of patients in clinical remission/endemic individuals.

Fig. 4. Lymphoproliferative, NO and cytokine responses of rLdiPGAM in cured patients/endemic contacts. Lymphoproliferative (A) and NO (B) response of PBMCs from individuals of Cured VL patients and Endemic controls (n = 7 for each group) against the recombinant proteins/SLD stimulation. The results were analysed by the one-way ANOVA test followed by Tukey's post test. Significance values indicate the difference between the SLD and the recombinant proteins’ stimulation P < 0·05(*), P < 0·01(**) and P < 0·001(***). (C) IL-12P40, IFN-γ and IL-10 level of PBMCs from individuals of cured VL patients and endemic controls against the recombinant proteins/SLD. All measurements were done in triplicate in two independent experiments and the results were comparable.
For the assessment of the Th1/Th2 stimulatory potential of the rLdiPGAM, the level of cytokines viz. IFN-γ, IL-12p40 and IL-10 were further estimated in the supernatant of stimulated PBMCs from infected and patients in clinical remission as well as in endemic and non-endemic individuals (Fig. 4C). IFN-γ and IL-12p40 levels were found to be higher in the supernatants of patients in clinical remission with a range of 348–751 and 746–2336 pg mL−1, respectively, followed by endemic controls (256–432 and 164–1417 pg mL−1, respectively), whereas very low level of IL-10 cytokine against rLdiPGAM was detected in the supernatants of patients in clinical remission (90–211 pg mL−1) followed by endemic controls (ranging from 53 to 231 pg mL−1). Thus, there was a shift exclusively towards Th1 type of immune response stimulated by rLdiPGAM as evident by the production of high amounts of IFN-γ and IL-12p40. There was suppression in the levels of IL-10, a Th1 deactivating cytokine, in patients with clinical remission as well as in endemic controls and a relatively low level of the same (as compared with SLD) in active cases. These findings indicate a precise and specific upregulation of Th1 stimulatory responses in patients in clinical remission with parallel downregulation of Th2 stimulatory responses.
Immunization with rLdiPGAM favours the development of Th1-type cellular responses in naive hamsters
To assess the immunogenicity of rLdiPGAM in modulating immune response of Th1/Th2 type, we further studied the status of iNOS transcript and Th1 (TNF-α, IFN-γ and IL-12p40) and Th2 [transforming growth factor beta (TGF-β), IL-4 and IL-10] in naive hamsters on day 30 post immunization. The level of iNOS transcript was observed to be upregulated by ~3 folds in immunized hamsters as compared with unimmunised controls. mRNA expression of Th1 cytokines, IFN-γ and IL-12p40, in rLdiPGAM immunized hamsters was observed to be significantly upregulated (P < 0·01 and 0·05, respectively) when compared with naive unimmunized controls. On the other hand, expression of Th2 cytokines, TGF-β and IL-10, was found to be downregulated on day 30 p.i. (P < 0·001 and 0·05, respectively) in the immunized animals (Fig. 5).

Fig. 5. Analysis of Splenic iNOS and cytokine mRNA expression profile by quantitative RT–PCR in normal and immunized hamsters on day 30 p.i. Results are presented as means and s.d. for five hamsters per group done in triplicate and are representative of two independent experiments with similar results. The analysis of the results was done by Student's t test. Significance values indicate the difference between the immunized group and normal group. P < 0·05(*), P < 0·01(**) and P < 0·001(***).
Vaccination with riPGAM generates considerably good prophylactic efficacy in hamsters against L. donovani challenges
Prophylactic efficacy of rLdiPGAM was further evaluated along with BCG as an adjuvant, in hamsters against L. donovani challenge. The vaccinated hamsters survived for a long duration post challenge and 67% (P < 0·001) of animals remained alive and healthy till 6 months p.c. when the experiment was terminated. On the other hand, hamsters vaccinated with BCG and unvaccinated infected control could survived only up to 2–3 months (Fig. 6).There was a gradual weight loss in the animals of the infected and BCG control groups whereas in the vaccinated animals a weight gain with time (P < 0·05 on day 45 p.c. and P < 0·01 on day 60 p.c.) was observed as noticed in normal hamsters. A remarkable reduction of hepatosplenomegaly was also observed in the vaccinated group, which is usually associated with the Leishmania infection (Fig. 7A–C). With an exception to the rLdiPGAM vaccinated group, an increase in parasite number from 3 × 103 to 4 × 103 was noticed in Giemsa-stained splenic dab smears of all the experimental hamsters from days 45 to 60 p.c. (Fig. 7D). In the vaccinated group, parasite loads decreased substantially from 2 × 103 on day 45 to 0·5 × 103 by day 120 p.c. Similarly, a decrease in parasite loads was seen in liver and bone marrow, after day 45 p.c. in the same vaccinated group (Fig. 7E and F).

Fig. 6. Survival curve analysis of different experimental groups. Survival data of animals (six hamsters in each group), representative of two independent experiments with similar results was observed up to day 180 p.c.

Fig. 7. Body weight, spleen weight and liver weight as well as parasite burden in rLdiPGAM + BCG vaccinated hamsters. Body weight (A), spleen weight (B) and liver weight (C) in g as well as parasite burden (no. of amastigotes per 10 001 cell nuclei) in the spleen (D), liver (E) and bone marrow (F) of hamsters on days 0, 45, 90, 120 and 180 p.c. Results are means and s.d. for three to four hamsters per group done in triplicate and are representative of two independent experiments with similar results. The analysis of the results was done by the one-way ANOVA test followed by Tukey's post test Significance values indicate the difference between infected group and other experimental groups P < 0·05(*), P < 0·01(**) and P < 0·001(***).
rLdiPGAM vaccination stimulates mitogenic, and Leishmania-specific cellular responses and alters Leishmania-specific IgG and its isotypes
rLdiPGAM vaccination in hamsters induces Con A-specific lymphoproliferative response similar to that observed in normal ones throughout the p.c. period (days 45 and 60), whereas it was lower in other control groups. A significant antigen-specific lymphoproliferative response (P < 0·001) was observed in the cells of rLdiPGAM vaccinated hamsters when compared with hamsters vaccinated with BCG alone and infected control group wherein a lesser proliferative response was observed (Fig. 8A and B). The supernatant of rLdiPGAM-stimulated lymphocytes from vaccinated hamsters produced remarkable levels of NO (~2·4-fold), which also supports the view regarding the upregulation of iNOS by Th1 cell-associated cytokines. Mouse J774A.1 macrophages line, when incubated with stimulated supernatants of lymphocytes from rLdiPGAM + BCG vaccinated hamsters, produced more significant (P < 0·001) amounts of NO (14·36 ± 2·18 µ m) than the other control groups (6·7–6·9 µ m values) on day 45 p.c., which further increased by day 60 p.c.(Fig. 8C and D).

Fig. 8. Lymphoproliferatve, NO and antibody responsed in rLdiPGAM vaccinated hamsters. (A, B) LTT response in Con A and rLdiPGAM stimulated lymphocytes of rLdiPGAM + BCG-vaccinated hamsters in comparison with the unimmunized infected hamsters on days 45 and 60 p.c. (C, D) NO production following LPS and rLdiPGAM stimulation in lymphocytes of rLdiPGAM + BCG-vaccinated hamsters in comparison to the unimmunized infected hamsters on days 45 and 60 p.c. (E, F) Leishmanial antigen-specific IgG and its isotypes IgG1 and IgG2 in rLdiPGAM + BCG vaccinated hamsters in comparison with the unimmunized infected hamsters on days 45 and 60 p.c. Data are presented as mean absorbance ± s.d. for three to four hamsters per group in triplicate wells and are representative of two independent experiments with similar results. The analysis of the results was done by the one-way ANOVA test followed by Tukey's post test Significance values indicate the difference between the vaccinated group and infected group P < 0·05(*), P < 0·01(**) and P < 0·001(***).
The leishmanial antigen-specific IgG and its isotypes (IgG1 and IgG2) in sera of all the experimental groups were assessed by ELISA. There was a progressive increase with time in the levels of anti-Leishmanial IgG and IgG1 in all the groups, except the rLdiPGAM + BCG vaccinated group, wherein the level significantly remained at lower levels than that of infected and BCG controls. In contrast, there was a significant elevation (P < 0·001) in the IgG2 level in rLdiPGAM + BCG vaccinated animals in comparison with the other experimental and control groups (Fig. 8E and F).
rLdiPGAM + BCG vaccination generates protective immune response of Th1 type in vaccinated hamsters
On days 45 and 60 p.c., the mRNA expressions of Th1 and Th2 cytokines i.e. TNF-α, IFN-γ, IL-12p40, TGF-β, IL-4 and IL-10 and iNOS were evaluated in rLdiPGAM + BCG vaccinated group and compared with L. donovani infected hamsters. There was a significant increase in the level of IL-12p40 at both the time points (P < 0·01 at day 45 and P < 0·05 at day 60 p.c.) as compared with the infected controls. The expression of iNOS and IFN-γ mRNA transcript levels also increased significantly on days 45 and 60 p.c. (P < 0·001). However, there was no significant elevation in TNF-α expression at both the time points (P > 0·05); whereas, on the other hand, extreme downregulation in the expression levels of TGF-β and IL-10 expressions was noticed in the vaccinated group on days 45 (P < 0·001) and 60 p.c. (P < 0·001 for TGF-β and P < 0·05 for IL-10) in comparison with the infected ones. However, the expression of IL-4 was not significant at all the time points (Fig. 9).

Fig. 9. Splenic iNOS and cytokine mRNA expression profile analysis of normal and vaccinated hamsters on days 45 and 60 p.c. by the qRT–PCR. Results are means and s.d. for three to four hamsters per group done in triplicate and are representative of two independent experiments with similar results. The results were analysed by the one-way ANOVA test followed by Tukey's post-test. Significance values indicate the difference between the vaccinated group and infected group P < 0·05(*), P < 0·01(**) and P < 0·001(***).
DISCUSSION
Regardless of a big repository of various vaccine candidates under different stages of development, no licensed vaccine is yet available against VL. A number of defined subunit vaccine candidates of several Leishmania species, are still at various experimental stages (Abdian et al. Reference Abdian, Gholami, Zahedifard, Safaee and Rafati2011; Bolhassani et al. Reference Bolhassani, Gholami, Zahedifard, Moradin, Parsi, Doustdari, Seyed, Papadopoulou and Rafati2011; Stober et al. Reference Stober, Jeronimo, Pontes, Miller and Blackwell2012). LEISH-F1 + MPL-s.e. is the only second-generation vaccine, which was assessed for its safety and immunogenicity (Chakravarty et al. Reference Chakravarty, Kumar, Trivedi, Rai, Singh, Ashman, Laughlin, Coler, Kahn, Beckmann, Cowgill, Reed, Sundar and Piazza2001) in India and is currently in clinical development for human VL (http://clinicaltrials.gov). As a step towards identifying novel and effective vaccine candidates against VL, cofactor-independent phosphoglycerate mutase (iPGAM) was identified as one of the probable Th1 stimulatory protein in the soluble sub-fraction of L. donovani (SLD) antigens ranging from 89·9 to 97·1 kDa (Gupta et al. Reference Gupta, Sisodia, Sinha, Hajela, Naik, Shasany and Dube2007; Kumari et al. Reference Kumari, Samant, Misra, Khare, Sisodia, Shasany and Dube2008b), in one of our previous studies.
The gene analysis of LdiPGAM demonstrated its homology to L. infantum and other Leishmania spp. to the tune of 90–94% (Supplementary Table S1). Moreover, when the relative expression of LdiPGAM was determined in different phases of cell cycle, there was maximum expression of protein in G1 and S phases and down expression in late S and G2/M phases, which supported the view that induction of glycolytic enzyme activities and increase of glycolytic rates during the G1 phase of the cell cycle are essential for cell process of RNA and DNA synthesis in the S phase (Brand et al. Reference Brand, Aichinger, Forster, Kupper, Neumann, Nurnberg and Ohrlsch1988). Bio-informatic approach led to the identification of two novel inhibitors of this protein molecule which, if explored, may further serve as drug candidates.
Since, LdiPGAM was recognized in immunogenic sub-fraction of SLD (Kumari et al. Reference Kumari, Samant, Khare, Sundar, Sinha and Dube2008a), we, further evaluated its immunogenicity, which showed that the recombinant protein stimulated better cellular immune response in treated Leishmania-infected hamsters as compared to SLD. Though, many studies have been conducted with SLD (Garg et al. Reference Garg, Gupta, Tripathi, Hajela, Sundar, Naik and Dube2006; Kumari et al. Reference Kumari, Samant, Khare, Sundar, Sinha and Dube2008a, Reference Kumari, Samant, Misra, Khare, Sisodia, Shasany and Dubeb), the response generated thereof was found to be partially protective and moreover cannot be specified due to the presence of multiple proteins whereas the immunogenic responses generated against rLdiPGAM are specific and better as compared to SLD. Moreover, major problems with the fractionated vaccines for human applications are their production to good clinical manufacturing standards, as well as gene variation and polymorphisms in field isolates. On the other hand, refined vaccines, such as recombinant proteins, have more feasible application for mass vaccination (Kumar and Engwerda, Reference Kumar and Engwerda2014).
We further evaluated the cellular immune response of the recombinant protein in hamsters in order to correlate the experimental observations with the human samples. Hamster serves as a good model of the systemic L. donovani infection as disease pathogenesis mimics to human Kala-azar patients (Melby et al. Reference Melby, Chandrasekar, Zhao and Coe2001). Herein, the immunogenicity of rLdiPGAM observed in hamsters, corroborated well in VL patients that were treated with amphotericin B as well as in endemic controls.
Further, since, it is well known that in case of leishmanial infection, macrophages, activated by IFN-γ released from parasite-specific T cells, are able to destroy intracellular parasites through the production of several mediators particularly NO (Liew et al. Reference Liew, Li, Moss, Parkinson, Rogers and Moncada1991; Assreuy et al. Reference Assreuy, Cunha, Epperlein, Noronha-Dutra, O'Donnell, Liew and Moncada1994). Herein, rLdiPGAM induced significantly higher proliferative responses and NO production in lymphocytes of treated Leishmania-infected hamsters as compared to normal as well as infected ones.
Since, it is a well-known fact that the recovery of individuals from leishmanial infection depends on the shifting of the immune response towards Th1 type (Belosevic et al. Reference Belosevic, Finbloom, Van Der Meide, Slayter and Nacy1989; Holaday et al. Reference Holaday, Sadick, Wang, Reiner, Heinzel, Parslow and Locksley1991), with the release of IL-12p40 and IFN-γ (MacMicking et al. Reference MacMicking, Xie and Nathan1997; Kumari et al. Reference Kumari, Samant, Khare, Sundar, Sinha and Dube2008a) the immunogenicity in terms of Th1 and Th2 cytokines being produced in response to rLdiPGAM was further assessed. The high level of IFN-γ and IL-12p40, and suppressed level of IL-10 in endemic control as well as in Leishmania patients in clinical remission indicate towards the immunogenic potential of this protein.
As a first step towards translating our data in vivo, the immunogenic potential of the rLdiPGAM was evaluated (post immunization) in naïve hamsters as compared with the unimmunized ones. The shifting of cellular immune response towards Th1 arm as indicated by the increased level of mRNA transcripts of iNOS and Th1 cytokines particularly IFN-γ further ascertained towards the potential of the protein in study as a novel vaccine candidate against VL.
The ability of rLdiPGAM to stimulate a strong Th1-type immune response encouraged us to assess its prophylactic efficacy along with BCG as an adjuvant, in hamsters against L. donovani challenge. BCG had been used in adjunct to several immunological agents (Khalil et al. Reference Khalil, El Hassan, Zijlstra, Mukhtar, Ghalib, Musa, Ibrahim, Kamil, Elsheikh, Babiker and Modabber2000; Misra et al. Reference Misra, Dube, Srivastava, Sharma, Srivastava, Katiyar and Naik2001), due to its ability to activate macrophages to generate NO for its microbicidal functions as reported by Armijos et al. (Reference Armijos, Weigel, Calvopina, Hidalgo, Cevallos and Correa2004) and Nozaki et al. (Reference Nozaki, Hasegawa, Ichiyama, Nakashima and Shimokata1997). Vaccination with rLdiPGAM + BCG have exerted considerably good prophylactic efficacy (73%) (Samant et al. Reference Samant, Gupta, Kumari, Misra, Khare, Kushawaha, Sahasrabuddhe and Dube2009; Gupta et al. Reference Gupta, Kushawaha, Tripathi, Sundar and Dube2012; Kushawaha et al. Reference Kushawaha, Gupta, Tripathi, Sundar and Dube2012) against L. donovani challenge in hamsters and approximately 67% of vaccinated animals could survived up to 6 months p.c. supported by a strong cell mediated immunity (CMI) response.
One measure among the several parameters of CMI, is mitogen and antigen-specific proliferation related to T-cell stimulation in vitro, which almost relates the control of parasite multiplication and healing in animals and humans (Melby et al. Reference Melby, Tryon, Chandrasekar and Freeman1998a, Reference Melby, Yang, Cheng and Zhaob; Armijos et al. Reference Armijos, Weigel, Calvopina, Hidalgo, Cevallos and Correa2004; Baharia et al. Reference Baharia, Tandon, Sahasrabuddhe, Sundar and Dube2014). Since, during active VL there is an impairment of T-cell proliferation (Kumar and Nylen, Reference Kumar and Nylen2012) the rLdiPGAM vaccination stimulated ~2-folds T-cell proliferation in hamsters after L. donovani challenge in comparison with the other experimental and control groups. However, since lymphoproliferation could not be regarded as the primary effector functions against the parasite, NO production by activated macrophages upon stimulation of Leishmania-specific T-cells has been considered to be the prominent and primary phenomenon of eliminating the intracellular parasite (Scott et al. Reference Scott, Pearce, Heath and Sher1987). In the present study also, a gradual increase in NO production (~2–3-folds) in the supernatant of macrophages co-stimulated with supernatant of rLdiPGAM-stimulated lymphocytes from vaccinated hamsters was noticed which also sustains the observation related to the upregulation of iNOS by Th1 cell-linked cytokines (Armijos et al. Reference Armijos, Weigel, Calvopina, Hidalgo, Cevallos and Correa2004). In addition, the vaccinated hamsters demonstrated higher IL-12p40 and IFN-γ cytokine levels along with remarkable downregulation of IL-10 and TGF-β as estimated by quantitative real-time PCR. The observation was well supported by the augmentation in the level of Leishmania-specific IgG2, which is also a sign of enhanced CMI (Kushawaha et al. Reference Kushawaha, Gupta, Sundar, Sahasrabuddhe and Dube2011).
In a nutshell, the rLdiPGAM, an enzyme of glycolytic pathway, is competent enough of inducing a strong cellular immune response in vitro as well as eliciting a moderate protective response against experimental VL in vivo in hamster model. Since, there is no effective vaccine as yet, the potential of this novel vaccine candidate could be validated and explored further for the optimization either alone or in combination with other potential vaccine candidates or adjuvants. Moreover, as a scope for more in-depth exploration, future study could be directed towards the identification and synthesis of immunodominant epitopes of this protein, which would generate more specific and robust T-cell response.
SUPPLEMENTARY MATERIAL
The supplementary material for this article can be found at https://doi.org/10.1017/S0031182017001160.
ACKNOWLEDGEMENTS
The authors are grateful to the Director of CDRI/CSIR, Lucknow for providing the facilities to make this study possible. The authors are thankful to Mr. A. L. Vishwakarma, SAIF, CDRI, Lucknow for providing Flow Cytometry study. This manuscript bears CSIR-CDRI communication no. 9513.
FINANCIAL SUPPORT
This work was supported by a grant from CSIR Network Project (HOPE-BSC0114) and Department of Biotechnology, New Delhi (BT/PR11782/MED/29/113/2009; Dated: 15.09.2009). Computational work was supported by grant from CSIR Network project GENESIS (BSC0121). The Council for Scientific and Industrial Research, New Delhi provided Junior (JRF-2 years) as well as Senior Research Fellowship (SRF-3 years) to R.T.
CONFLICT OF INTEREST
None.