Introduction
Migraine and other headache disorders have become the second most common disease globally, and are the second highest cause of disability worldwide.1 Despite the global impact, migraine’s pathogenesis is still not fully understood. A current prevailing theory of migraine pathogenesis is the hyperexcitable “trigeminovascular complex.” In this theory, cortical spreading depression starts a cascade of events leading to the release of neuropeptides such as calcitonin gene-related peptide and substance P from trigeminal nerve endings in the dura. This process results in neurogenic inflammation at the level of the meninges and excites the sensory trigeminal afferents. The meningeal sensory afferents enter the brain stem via the trigeminal tract and terminate on the spinal trigeminal nucleus. That information is transmitted then to multiple cortical areas and perceived as pain as well as other migraine symptoms, such as sensory and visual disturbances.Reference Noseda and Burstein2 Despite our increased understanding of migraine pathophysiology over the last several years, many details still remain unclear and the pathogenesis requires further study, particularly in pediatrics. As occurs with many conditions, much of our knowledge is from adult populations, and this knowledge is presumed to translate to pediatrics. However, children and adults do not experience migraines in the same way. Children, compared to adults, tend to experience migraines of shorter duration, with quick onset and offset. Children also have more prominent gastrointestinal symptoms with their migraines such as nausea and vomiting.Reference Bigal and Lipton3 Beyond differences in symptomology between children and adults, there are also alterations in migraine expression that occur throughout development; for example, puberty is often a time of transition: some children who suffered migraines stopped having them after puberty, whereas some adolescents developed migraines with puberty.Reference Congdon and Forsythe4–Reference O’Brien and Cohen6 Additionally, the ratio of migraineurs in males and females is equal before puberty and is more frequent in women in adulthood.Reference Guidetti, Alberton, Galli and Salvi7 These alterations in expression demonstrate that differences exist between pediatric migraine and adult migraine; therefore, one approach to better understand migraine is to examine the condition in children in comparison with adults.
Neuroimaging provides a mechanism to study neurological disorders. Investigation of the imaging similarities and differences between adult and child migraine patient populations offers three benefits. First, it characterizes the differences between pediatric and adult migraine. By recognizing that there are differences between the manifestation of this disorder in these two populations, researchers and clinicians recognize that there is a need to tailor therapies depending on the age. This is particularly relevant in light of the recent finding that preventative medications (Amitriptyline and Topiramate) that are standard practice for adult migraine reduce headache days in children but not more than placebo therapy.Reference Powers, Coffey and Chamberlin8 Second, examining migraine across the lifespan may assist to address issues of biomarkers versus byproduct. As children have had migraines for a shorter period, comparisons between children and adults offer a unique avenue to explore whether traits seen in adult migraine are causal or a result of migraine.Reference Bigal and Arruda9 For example, it is unclear whether white matter lesions (WML) indicate a predisposition to severe migraines, or if these appear as a result of migraines. Understanding this biology is important for developing therapies. Third, because not all pediatric migraine patients continue to have migraines as adults,Reference Congdon and Forsythe4–Reference O’Brien and Cohen6 and puberty can be a time to develop migraines,Reference Guidetti, Alberton, Galli and Salvi7 understanding the differences between adult and childhood migraine provides the opportunity to identify developmental changes that increase (or decrease) susceptibility to migraine.Reference O’Brien and Cohen6
Currently, pediatric migraine is understudied, particularly considering the potential individual and societal benefits of a thorough characterization of pediatric migraine. This review, therefore, aims to motivate further research in the field to improve this reality, and suggested research paths are presented.
Magnetic resonance imaging (MRI) is a noninvasive imaging method with no ionizing radiation. It is clinically safe for children and provides a tool for researching the brain. Neurosciences research has greatly benefited from MRI, as different acquisitions can characterize multiple aspects of physiology in vivo. Here, our aim is to review the current understanding of pediatric migraine using MRI modalities. This literature review briefly overviews applicable MRI methods relevant for migraine research (structural imaging, functional connectivity, spectroscopy, and perfusion) and compares MRI findings in these modalities in childhood migraine with results from adults. Amalgamating MRI data is important as different MRI modalities offer insight into specific aspects of brain function and together give a more complete picture of the underlying nature of migraine.
White Matter Structure
Conventional diagnostic MRI is used to detect gross structural abnormalities. T2-weighted imaging, a standard clinical pulse sequence, is often used to highlight white matter (WM) hyperintensities (WMH). WMH in migraine have been well documented.Reference Hamedani, Rose and Peterlin10–Reference Park, Lee, Kim, Yun and Kim13 A central question regarding WM changes in migraine is whether these are a biomarker of susceptibility or byproduct of the disease.
WMH have been widely studied in relation to cognitive impairment, stroke, and dementia.Reference Debette and Markus14 and are often presumed to have a vascular origin.Reference Wardlaw, Valdés Hernández and Muñoz-Maniega15 While WMH have been widely studied, different quantification methods (e.g., automated detection, visual inspection, and volume determination) exist. In the pediatric population, there is no consensus on measurement methods. Migraine also has associations with cognitive impairment,Reference de Araújo, Barbosa, Lemos, Domingues and Teixeira16 stroke,Reference Kurth, Chabriat and Bousser17 and dementia.Reference Chuang, Lin, Lin, Sung and Kao18 WMH are often interpreted as demyelination and axonal injury, which may be an underlying problem in migraine.Reference Fazekas, Kleinert and Offenbacher19 Several adult studies show WMH are more common in migraineurs than in control populations, and WMH have been shown to be progressive in migraineurs.Reference Palm-Meinders, Koppen and Terwindt12, Reference Erdélyi-Bótor, Aradi and Kamson20 Specifically, a 9-year follow-up study showed adults with migraine developed significantly more WMH than the control population.Reference Palm-Meinders, Koppen and Terwindt12
While the documented progressive nature of WMH in migraine suggests that WMH are a byproduct of migraine, WMH are also found in children with migraine, which may indicate otherwise.Reference Hämäläinen, Autti, Salonen and Santavuori21, Reference Yılmaz, Çeleğen, Yılmaz, Gürçınar and Ünalp22 Studies assessing WMH in children often focus on the more severe WML, a potential bias in the literature. WML are a WMH that are caused by a degradation of myelin, whereas WMH may also have other origins such as normal structural variations, including lacunae or perivascular spaces. The prevalence rates of WML in childhood migraine patients are 6–67%Reference Candee, McCandless, Moore, Arrington, Minich and Bale23–Reference Bayram, Topcu, Karaoglu, Yis, Cakmakci Guleryuz and Kurul27 (Table 1), and WML in populations as young as 6 yearsReference Candee, McCandless, Moore, Arrington, Minich and Bale23 may suggest that WML are not a byproduct of chronic life-long migraines. One study suggests WML development is related to migraine frequency compared to a cumulative lifetime risk.Reference Eidlitz-Markus, Zeharia, Haimi-Cohen and Konen24 However, there are discrepancies in data regarding the prevalence and frequency of WML in relation to pediatric migraines. WML are also found in control populations of children, sometimes at prevalence rates of 4%, which may not significantly differ from pediatric migraine patients.Reference Mar, Kelly, Isbell, Aung, Lenox and Prensky25 There are two major limitations regarding these childhood migraine WMH studies. First, there is little consensus of how to accurately measure and quantify WMH in pediatric populations. Second, the results are based on retrospective chart analysis as opposed to prospective studies. As neuroimaging is not routinely done in migraine, these results are likely from more severe cases of migraine.
Table 1. Pediatric migraine white matter studies

Advanced imaging methods can interrogate brain anatomy and physiology. Particularly relevant to WM is diffusion tensor imaging (DTI). The diffusion signal is based on free diffusion of water, which is hindered by WM fibers.Reference Tamnes, Roalf, Goddings and Lebel28 DTI data enable maps depicting (a) the overall level of diffusion, typically shown as mean diffusivity (MD) and (b) the level of diffusion anisotropy or directionality associated with diffusion. Diffusion anisotropy is measured as: axial diffusivity (AD), the level of diffusion parallel to a WM tract; radial diffusivity (RD), diffusion across or perpendicular to the WM tract; and fractional anisotropy (FA), a composite where 1 is completely anisotropic diffusion (single orthogonal direction of diffusion) and 0 represents completely isotropic diffusion (Figure 1). Each of these metrics provides complementary information about WM structural integrity. For example, MD decreases with cytotoxic edema and increases with cell death;Reference Alexander, Hurley and Samsonov29 RD can index axonal diameter and increases with demyelination;Reference Alexander, Hurley and Samsonov29 AD values decrease with axonal injuryReference Alexander, Hurley and Samsonov29, Reference Sun, Liang, Le, Armstrong, Cross and Song30 and increase with brain maturation;Reference Alexander, Hurley and Samsonov29, Reference Ashtari, Cervellione and Hasan31–Reference Gao, Lin and Chen33 and more aligned tracts show larger FA values, and disruptions in WM tracts cause decreases in FA.Reference Alexander, Hurley and Samsonov29 FA also increases with age and is a marker of brain maturation; it increases from 10% to 25% between the ages of 5 and 25 years.Reference Lebel and Deoni34, Reference Lebel, Walker, Leemans, Phillips and Beaulieu35
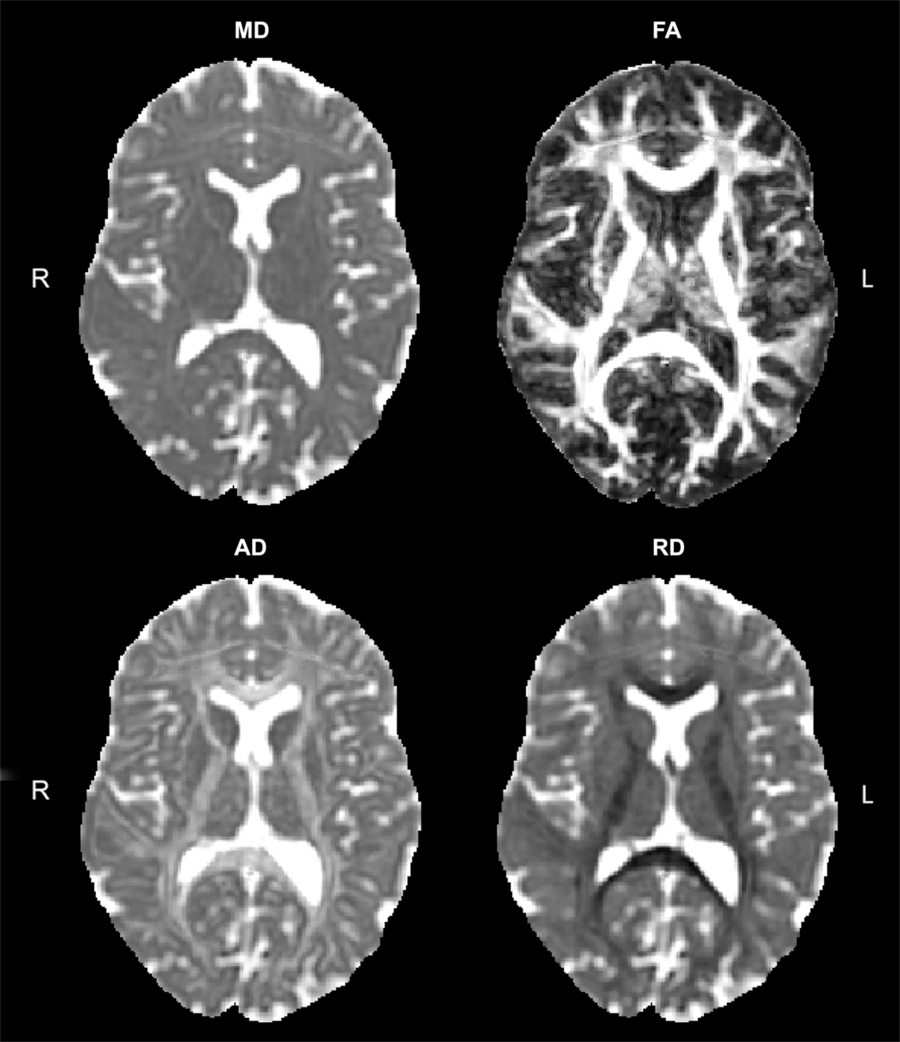
Figure 1: Sample DTI maps.
To date, only one study has used DTI in childhood migraine (Table 1).Reference Messina, Rocca and Colombo36 The study found decreases in MD, RD, and AD in the corpus callosum, cingulum, corticospinal tract, and superior longitudinal fasciculus (nociceptive pathways), indicating WM tract disruption in the absence of any WMH.Reference Messina, Rocca and Colombo36 Similarly, the optic tract and optic radiations also showed decreased MD, RD, and AD but increased FA. As this study did not differentiate between migraine with aura (MA) and migraine without aura (MO), this may be an area for future studies to compare. From this single study, the authors were unable to conclude whether WM abnormalities stemmed from overuse because migraine experience or prior alteration of these tracts predisposes patients to migraine. While this study did have an age-matched control group, brain development and maturation is an important consideration in interpreting this data.
Some adult studies using DTI techniques show demyelination and axonal injury in the nociceptive pathways and migraine-related areas, though other studies show disruptions in different regions.Reference Chong and Schwedt37–Reference Rocca, Pagani and Colombo39 One study found demyelination in the left corticospinal tract, the right inferior longitudinal fasciculus, and the anterior thalamic radiations, but no group differences in FA values.Reference Chong and Schwedt37 Another study found demyelination and axonal injury in the corpus callosum, and the right anterior and posterior limb of the internal capsule.Reference Yu, Yuan and Qin38 A final study found demyelination in the visual pathway with reduced FA values compared to controls.Reference Rocca, Pagani and Colombo39 All these studies show decreased WM tract density in pathways associated with migraine, contrasting observations in pediatric migraine (Table 1).
There are discrepancies in DTI results in the nociceptive pathways between pediatric and adult migraineurs. However, it is important to consider that myelination and therefore DTI metrics change over the lifetime; and in particular, pronounced changes of DTI metrics occur throughout development.Reference Bava, Thayer, Jacobus, Ward, Jernigan and Tapert32, Reference Gao, Lin and Chen33 As such, contrasts between these studies need to be interpreted cautiously. In pediatric migraine, results show the nociceptive pathway are denser with greater axonal injury compared to controls.Reference Messina, Rocca and Colombo36 In adults, DTI data have shown the opposite, with results indicating demyelination.Reference Chong and Schwedt37 This is potentially an interesting finding for future research as it could be a surrogate imaging marker in migraine and should be further validated. If nociceptive pathways are injured in pediatric migraine, decreased MD, RD, and AD may indicate inflammation. In adult migraine patients, increased MD, RD, and AD suggests axonal injury and demyelination. Inflammation in pediatric migraine may progress to axonal injury in adult migraine and may explain the transition differences in migraine, though normal developmental trajectories confound these investigations. Data for these conclusions are, however, based on limited pediatric evidence, and further studies using DTI in pediatric migraine would be important to support what is reviewed here.
Gray Matter
Conventional anatomical MRI, typically T1-weighted or T2-weighted imaging, have been used to investigate gray matter. This imaging provides detailed structural information, enabling morphometric measurement of structures directly, or more complex analysis can investigate cortical thickness and gray matter density.
In pediatric migraine, gray matter structure has been less studied than WM; to date, there are only three studies looking at gray matter structure in pediatric migraine (Table 2), all using different methods and examining different brain areas.
Table 2. Pediatric migraine gray matter studies

The brainstem, and in particular the periaqueductal gray matter (PAG), has long been theorized as a migraine generator.Reference Rocca, Ceccarelli and Falini40, Reference Weiller, May and Limmroth41 A study comparing the diameter of the brainstem in the coronal and midsagittal planes in childrenReference Hämäläinen, Autti, Salonen and Santavuori21 has found the diameter of the pons to be significantly greater in the migraine group compared with age- and sex-matched controls, although still within the normal range. No differences were seen in the diameter of the medulla oblongata or the midbrain. The authors argued this result supports the theory that the brainstem acts as a migraine generator, and its overactivity and neurogenic inflammation contribute to the slightly larger size of the pons in pediatric migraineurs compared with controls;Reference Hämäläinen, Autti, Salonen and Santavuori21 however, this result is limited as it is based on a single measure of the diameter, not a volumetric assessment. Changes in brainstem gray matter have also been observed in adult migraine, specifically, increased gray matter density in the PAG as well as increased gray matter density of the dorsolateral pons in adult patients with MA compared with controls.Reference Rocca, Ceccarelli and Falini40 More recently, diffusion kurtosis imaging (DKI) was used to investigate PAG in adult migraine. DKI is an extension of DTI that aims to assess non-Gaussian components of diffusion and is used to study gray matter. Higher mean kurtosis (MK) indicates diffusional heterogeneity, and MK increases tend to be associated with cell density or tissue complexity.Reference Zhuo, Xu and Proctor42 This study showed increased MD and increased MK values (both indicative of increased cell density) in the periaqueductal gray matter in patients, and MK was correlated with age and duration of disease without treatment.Reference Ito, Kudo and Sasaki43 Consistencies between adults and pediatrics in migraine presentation demonstrate hallmarks of the disorder or aspects that are affected early and remain throughout the lifetime. In both pediatrics and adults with migraine, there is evidence of brainstem gray matter increases. This early evidence of brainstem increases lends evidence to the theory that the brainstem is highly involved in migraine pathophysiology and suggests that brainstem changes are characteristic of the disorder (Table 2).
Beyond the brainstem, a second study using a more global approach to investigate gray matter changes found widespread differences in pediatric migraine patients (ages 9–18).Reference Rocca, Messina, Colombo, Falini, Comi and Filippi26 Compared to age-matched controls, migraine patients showed significantly less gray matter density in the frontal and temporal lobes. Interestingly, these reductions in gray matter were not correlated with disease duration or attack frequency. Adult migraine studies have also demonstrated decreased gray matter density and decreased cortical volume in the frontal cortex of migraineurs compared with controls.Reference Dai, Zhong and Xiao44–Reference Schmitz, Admiraal-Behloul and Arkink47 Components of the pain-processing network are located in the frontal cortex; thus, it has been suggested that frontal cortex atrophy is related to the reorganization of the pain network as a result of migraine,Reference Hougaard, Amin, Magon, Sprenger, Rostrup and Ashina45 which is supported by the demonstration of atrophy in other parts of the pain network such as the subgenual cingulum.Reference Rocca, Messina, Colombo, Falini, Comi and Filippi26 Alternatively, frontal atrophy may be a biomarker of migraine,Reference Rocca, Messina, Colombo, Falini, Comi and Filippi26 which is supported by the fact that, in both children and adults with migraine, decreased gray matter volume in the frontal cortex is not associated with headache frequency or duration.Reference Kim, Suh and Seol46–Reference Messina, Rocca and Colombo48 The frontal cortex atrophy seen in migraine patients is an especially important aspect of pediatric migraine disorder. Adult migraine patients have an elevated risk of cognitive dysfunction with complaints regarding attention and memory,Reference de Araújo, Barbosa, Lemos, Domingues and Teixeira16 but similar findings have also been seen in children and adolescents (age 10–18 years).Reference Costa-Silva, de Prado, de Souza, Gomez and Teixeira49 The connection between frontal cortex atrophy and executive function deficits is obvious and warrants further investigation. One important question to answer would be which comes first, the frontal atrophy or migraine. If migraine is the cause of frontal atrophy resulting in cognitive deficits, then preventing or limiting migraine in pediatrics becomes critically important. Investigating migraine in younger children, or in children before the onset of migraine, in a longitudinal study would be an interesting avenue to explore this question.
Gray matter in the temporal lobe was affected differently in pediatric patients with and without migraine aura. In MA patients, the left fusiform gyrus had greater gray matter volume compared with controls and MO patients. Also MO patients showed reduced volume of the fusiform gyrus compared with controls. Aura in migraine is a visual disturbance. Because the fusiform gyrus contributes to high-order visual processing, a difference between MA and MO patients is not surprising.Reference Rocca, Messina, Colombo, Falini, Comi and Filippi26 The increased size of the fusiform gyrus in pediatric MA patients may be related to inflammation, though it is unclear why there would be decreased volume in pediatric MO. Interestingly, this exact finding has either not been studied or not been demonstrated in adults, and a recent meta-analysis showed no evidence of changes in that area.Reference Jia and Yu50 It is possible based on the large volume of investigations of gray matter in adult migraine patients and the lack of evidence demonstrating that this is a purely pediatric phenomenon and may be partially responsible for the variation in migraine presentation between adults and children.
A recent study investigating gray matter in pediatric migraine examined the interactions of sex and age on whole-brain gray matter cortical thickness, comparing migraine patients and controls (Table 2).Reference Faria, Erpelding, Lebel, Johnson, Wolff and Fair51 Cortical thickness differences between female and male migraine patients appeared to be an important distinction to investigate. Compared with males, females have greater gray matter cortical thickness in the primary somatosensory cortex. Female adolescents with migraine had greater cortical thickness compared with male adolescents with migraine and all healthy controls.Reference Faria, Erpelding, Lebel, Johnson, Wolff and Fair51 Interestingly, in adults with migraine, there have been conflicting results with studies demonstrating increased somatosensory cortical thickness,Reference DaSilva, Granziera, Snyder and Hadjikhani52 no difference in thickness of the somatosensory cortex in migraine patients,Reference Datta, Detre, Aguirre and Cucchiara53 and also thinning of the somatosensory cortex in migraine patients.Reference Hougaard, Amin and Arngrim54 Somatosensory cortical thickness in female adults appears to be important, however, as somatosensory cortical thickness is correlated negatively with response to medications for migraines.Reference Hubbard, Becerra and Smith55 Female children and adolescents in general also had greater cortical thickness in areas associated with nociception, including the precuneus, supplementary motor area, basal ganglia, and amygdala.Reference Faria, Erpelding, Lebel, Johnson, Wolff and Fair51 The authors found sex × disease interactions in structural findings that indicated females with migraine have significant gray matter thickening in the right supplementary motor area, and right precuneus compared with males with migraine and healthy controls.Reference Faria, Erpelding, Lebel, Johnson, Wolff and Fair51 Female adults have also been found to have more gray matter in the precuneus compared with male adults with migraine and controls.Reference Maleki, Linnman, Brawn, Burstein, Becerra and Borsook56 Precuneus findings in females are especially relevant, as the precuneus has been correlated with pain sensitivity in adults.Reference Goffaux, Girard-Tremblay, Marchand, Daigle and Whittingstall57 Baseline pain sensitivity is an important measure as it is closely related to the risk of developing chronic pain.Reference Granot58–Reference Strulov, Zimmer, Granot, Tamir, Jakobi and Lowenstein61 As migraine is a form of chronic pain, precuneus data indicate that increased size may be a risk factor for experiencing migraine. Overall, these findings indicate that sex differences in migraine are important and demonstrate that sex differences in gray matter volumes of pain-related areas in the brain might predispose females to developing migraine or being less responsive to medication.
Perfusion
Perfusion imaging aims to depict and quantify tissue perfusion and can be quantified in terms of cerebral blood flow, amount of blood passing through a tissue capillary bed (quantified in units of mL/100 g tissue per minute), cerebral blood volume, average volume of blood within a tissue bed (mL/100 g tissue), or mean transit time, that is, time (per second) taken by the blood to pass through a tissue. Perfusion can be measured with MRI using (a) dynamic susceptibility contrast, in which a venous injection of contrast agent is administered and its passage through tissue is imaged to determine perfusion characteristics or (b) arterial spin labeling (ASL) in which blood is magnetically tagged and the passage of the magnetically tagged blood compared to imaging without magnetic tagging is subtracted to reveal perfusion maps. For research studies, ASL is more appealing as it does not require any injections. Clinically, dynamic susceptibility imaging is often used, or other imaging modalities, such as single photon emission computed tomography (SPECT), have also been applied.
Perfusion in pediatric migraine has most commonly been described through case studies of hemiplegic migraine, a rare form of MA involving motor weakness. By their nature, it is difficult to draw conclusions from case studies, but these can show trends when taken in aggregation. In the case of pediatric hemiplegic migraine, there is a trend of transient hypoperfusion contralateral to the side of aura (Table 3).Reference Altinok, Agarwal, Ascadi, Luat and Tapos62–Reference Toldo, Cecchin and Sartori66 While inconsistencies in this trend have been reported,Reference Kumar, Topper and Maytal67 factors such as perfusion being measured multiple days after onset of symptoms confound these results. Consistent with these single case studies of altered contralateral perfusion, a small study (n = 4) using susceptibility-weighted imaging (SWI) showed asymmetry in the right and left cerebral vasculature in the early stages of hemiplegic migraine (within 6 h of symptom onset) with abnormalities in SWI contralateral to the hemiparesis, which resolved in follow-up SWI assessments.Reference Fedak, Zumberge and Heyer68 While SWI is not designed to detect tissue perfusion, it is highly sensitive to iron deposition, microbleeds, and vasculature. A larger (n = 10) case–control study showed that cerebral blood flow decreased in brain regions associated with aura symptoms when MRI was performed <14 h after onset and increased if MRI was performed ≥17 hours after onset. Time course alterations were, however, not linked with the persistence of aura symptoms.Reference Boulouis, Shotar and Dangouloff-Ros69 Using ASL and MR angiography in retrospective chart analysis, it was found that in pediatric patients with MA, there was homolateral hypoperfusion of the side of vasospasm.Reference Cadiot, Longuet and Bruneau70 A more recent study also using ASL found differences between pediatric migraine patients with and without perfusion changes during migraine. This study has found that patients who had perfusion abnormalities were more likely to have aura, motor disabilities, confusion, and hospitalization. In this study, however, there were differences in MRI application (24 h, 6 days, and 7 days) between participants, which could have altered the findings.Reference Uetani, Kitajima and Sugahara71 The type of migraine may also influence observations in perfusion imaging; the last two studies investigated atypical MA, whereas all the previously mentioned case studies examined familial hemiplegic migraine (Table 3). Adult literature shows regional hypoperfusion during the aura phase of migraine and hyperperfusion during the headache phase,Reference Friberg, Olesen, Lassen, Olsen and Karle72–Reference Pollock, Tan, Kraft, Whitlow, Burdette and Maldjian76 consistent with the above findings in children.
Table 3. Pediatric migraine perfusion studies

Both adults and children with migraine appear to have differences in perfusion between migraines (interictally) as well during episodes (ictally). A recent study investigating pediatric and adult migraines has found increased blood flow to the somatosensory cortex in the interictal period of migraine as measured by ASL.Reference Youssef, Ludwick and Wilcox77 These findings mirrored earlier findings in adults that demonstrated increased cerebral blood flow (measured using ASL) in the primary somatosensory cortex in MO patients compared with controls. Primary somatosensory cerebral blood flow was also correlated with migraine frequency.Reference Hodkinson, Veggeberg and Wilcox78 These observations may explain increased sensory sensitivity in migraineurs and/or may indicate adaptive or maladaptive changes in the sensory cortex to explain migraine pain. The increase in blood flow to the somatosensory cortex may be constant as no differences in blood flow were seen between and during a migraine attack within the patient group.Reference Gil-Gouveia, Pinto, Figueiredo, Vilela and Martins79 These findings indicate that differences in blood flow are likely chronic and not attack-dependent. The correlation between pediatric and adult data also suggests that increased blood flow in the somatosensory cortex is a feature of migraine as opposed to a consequence of chronic migraine.
As perfusion investigation techniques improve, particularly with the more widespread use of ASL, it is expected that more studies will investigate perfusion differences between migraineurs and controls. This will assist in investigating hypotheses underlying migraine, including perfusion response to cortical spreading depression. Currently, the available literature suggests that perfusion differences are still a valuable avenue for exploration in the realm of migraine and deserve further investigations in pediatric migraine particularly.
Metabolites
Magnetic resonance spectroscopy (MRS) provides a method to measure the concentration of metabolites in localized volumes of tissue. Different target nuclei enable the quantification of different metabolites, the most typical of which are 1H followed by 31P MRS. Metabolite measurements give a different insight into brain structure, function, and metabolism. For example, metabolites can inform us about energy utilization, WM degradation, and the activity of neurotransmitters.
There are only two studies that investigated brain metabolites in childhood migraine (Table 4). The first study has found that the relative rate of mitochondrial oxidation, as determined by low levels of phosphocreatine concentration and high cytosolic pH, was higher in the occipital lobes of patients than in controls. They also found a lower phosphorylation potential in the brains of migraine patients, and that muscle mitochondrial respiration was abnormal. The authors concluded that there is a bioenergetics deficit in young migraine patients, which is possibly a defining feature of the disorder.Reference Lodi, Montagna and Soriani80 This bioenergetics deficit is consistent with findings in adult studies where phosphocreatine levels in the occipital lobe were reduced in patients with migraine, which may suggest mitochondrial dysfunction.Reference Barbiroli, Montagna and Cortelli81–Reference Welch, Levine, D’Andrea, Schultz and Helpern87 The same study also showed a 25% reduction in magnesium ion concentration in juvenile migraine patients, and decreased magnesium levels have been associated with an increased odds of having a migraine attack.Reference Welch, Levine, D’Andrea, Schultz and Helpern87, Reference Younis, Hougaard, Vestergaard, Larsson and Ashina88 Decreased serum levels of magnesium in adult migraine populations have previously been suggested as an independent risk factor for migraine attacks,Reference Assarzadegan, Asgarzadeh, Hatamabadi, Shahrami, Tabatabaey and Asgarzadeh89 and three adult studies have also found decreased brain magnesium in migraineurs in the occipital lobe,Reference Lodi, Iotti and Cortelli90 the anterior-posterior region,Reference Boska, Welch, Barker, Nelson and Schultz91 and the frontal and temporal lobes.Reference Ramadan, Halvorson, Vande-Linde, Levine, Helpern and Welch92 As such, decreased magnesium ion levels have been suggested to contribute to reduced mitochondrial oxidation as well as reduced bioenergetics seen in patients with migraine, as magnesium is a cofactor in oxidative phosphorylation and stabilizes the mitochondrial membrane.Reference Younis, Hougaard, Vestergaard, Larsson and Ashina88, Reference Welch and Ramadan93 In two double-blinded, placebo-controlled adult trials, oral magnesium was effective as a prophylactic treatment for migraine.Reference Peikert, Wilimzig and Köhne-Volland94, Reference Facchinetti, Sances, Borella, Genazzani and Nappi95 A third study did not see the same effects, but this was suggested to be due to the absorbability of the magnesium supplement.Reference Pfaffenrath, Wessely and Meyer96 The sole pediatric study saw a reduction in headache days and headache intensity using oral magnesium.Reference Wang, Lirng, Fuh and Chen97 Evidence suggests that magnesium may be an effective supplement for pediatric migraine patients. As pediatricians and neurologists seek treatments appropriate for migraines in adolescents, magnesium offers an area of research that should be further pursued, as it has good tolerability and minimal side effects.
Table 4. Pediatric migraine metabolites studies

The second study to investigate metabolites in pediatric migraine was a case study, which applied proton MRS (Figure 2) in a case of hemiplegic migraine 15 days after the migraine (Table 4). The authors found a decreased N-acetylasparatate (NAA)/creatine ratio in conjunction with contralateral hemisphere swelling and a mild hyperintensity visible on diffusion-weighted imaging.Reference Toldo, Cecchin and Sartori66 NAA is a neuronal marker, and reduction in NAA is often interpreted as decreased neuronal integrity (though this interpretation is incomplete; for a detailed review, see ref. Reference Rae98). Creatine provides a measure of energy stores. A single case study is not convincing to argue that this is typical of pediatric migraineurs, although several studies of migraine in adults have shown decreased NAA in various regions of the brain;Reference Dichgans, Herzog, Freilinger, Wilke and Auer99–Reference Zielman, Teeuwisse and Bakels103 one study observed an increase in NAA in the pons in episodic migraineurs,Reference Lai, Fuh, Lirng, Lin and Wang104 and many studies have not observed any differences in NAA levels in various regions of the brain.Reference Reyngoudt, Paemeleire, Descamps, De Deene and Achten84, Reference Schulz, Blamire, Corkill, Davies, Styles and Rothwell85, Reference Wang, Lirng, Fuh and Chen97, Reference Becerra, Veggeberg and Prescot105–Reference Watanabe, Kuwabara, Ohkubo, Tsuji and Yuasa114 Location, in addition to type of migraine, and migraine severity may also confound results and explain some of these discrepancies. A recent review argued that, instead of indicating neuronal loss, these reductions in NAA could indicate mitochondrial dysfunction, as mitochondria are proposed to synthesize NAA.Reference Younis, Hougaard, Vestergaard, Larsson and Ashina88 If the pediatric case study is replicated and generalized, however, it would lend evidence to mitochondrial dysfunction as one of the mechanisms involved in migraine pathogenesis and could also perhaps provide further support for magnesium supplementation.

Figure 2: Sample proton MRS.
Functional Imaging
Blood oxygen level-dependent (BOLD) functional MRI (fMRI) is an extensively used method to examine correlations in brain activation, either with a task paradigm (task-fMRI) or within the brain at rest (resting-state fMRI). Task-fMRI is generally used to examine brain activation in response to a stimulus, while resting-state fMRI examines functional connections and coherent activation in the absence of a task. Despite the widespread use of BOLD-fMRI to investigate alterations in functional activation and functional connectivity, only one BOLD-fMRI study has been performed in pediatric migraine (Table 5).Reference Faria, Erpelding, Lebel, Johnson, Wolff and Fair51 The analysis included dichotomizing participants by sex. Results using fMRI showed differences in resting-state functional connectivity (rsFC) between females with migraine compared with male patients and healthy controls. Females with migraine exhibited greater rsFC in the pain network, specifically between the right precuneus and the left putamen, right caudate, left thalamus, and left amygdala compared with males with migraine and healthy controls. Female patients also showed greater rsFC between the left amygdala and the bilateral thalamus, right supplementary motor area, and bilateral anterior midcingulate cortex compared with male patients and healthy controls. Studies on adults with migraine also showed increased connectivity in pain-processing networks. For example, a study applying graph theory analysis to fMRI data showed abnormal connectivity nodes in female adult migraine patients in the precentral gyrus, orbital part of the inferior frontal gyrus, parahippocampal gyrus, anterior cingulate gyrus, thalamus, temporal pole of the middle temporal gyrus, and the inferior parietal gyrus.Reference Liu, Zhao and Li115 Other literature in adults found similar results with greater connectivity in migraineurs in the orbital frontal gyrus, medial frontal cortex, inferior frontal cortex, insula, supplementary motor area, precentral gyrus, postcentral gyrus, inferior parietal gyrus, and occipital cortex.Reference Liu, Zhao and Li115, Reference Liu, Zhao and Lei116 Also, greater amygdala connectivity to the visceroceptive insula in migraine patients was seen compared with controls and other chronic pain groups.Reference Hadjikhani, Ward and Boshyan117, Reference Chen, Chen, Liu, Dong, Ma and Yu118 However, not all adult studies have found similar results. One study has found decreased functional connectivity in pain-related regions of the brain in female adult migraine patients, with lower functional connectivity in the bilateral hippocampus, bilateral insula, right amygdala, right anterior cingulate cortex, bilateral putamen, bilateral caudate nucleus, and prefrontal cortex.Reference Gao, Xu and Jiang119 Overall, the underlying state of connectivity is not clear, particularly with heterogeneous methods and analyses. What can be concluded is that abnormal connectivity changes in the pain network underlie the migraine condition; how those changes manifest appears to generally trend towards greater connectivity in the pain network in migraine patients, but further studies are required to confirm these findings.
Table 5. Pediatric migraine functional imaging studies

Discussion
MRI provides a great opportunity to understand neurological conditions, including pediatric migraine. However, very few pediatric migraine studies have applied MRI and spectroscopy; results are from case reports and small sample sizes. Thus, all findings need to be interpreted with caution. Beyond the limited data, there is also inherent heterogeneity within the pediatric population due to brain development as well as from a generalized lack of consensus in standardizing measurements from various MRI modalities. Seven interesting findings that require further research have come from this literature review. First, WM disruptions appear to be progressive in migraine with severity and chronicity. These WM changes require further study to delineate their correlation with neurological dysfunction. At this point, we do not have a way of addressing or preventing these other than by optimizing migraine treatments. Second, brainstem changes seen on imaging indicate a role in the pathophysiology of migraine in pediatrics, which correlates with adult findings. Third, decreased grey matter density in the frontal cortex in pediatric migraine patients may be an issue that needs to be addressed early, as it may be a factor for potential cognitive dysfunction at a later age. Fourth, increases in temporal or fusiform gyrus in gray matter are seen only in pediatrics, which identifies a uniquely pediatric aspect of migraine and is possibly connected to the varied presentation between pediatric migraine and adult migraine. Fifth, the precuneus is larger in female adolescents, especially in those with migraine. The role of the precuneus in pain sensitivity and risk of developing chronic pain conditions is a reasonable possible cause for sex differences in migraine prevalence in adulthood. The precuneus was not found to have a role in childhood migraine, although it is possible that changes in the precuneus during puberty may explain the prevalence of sex differences seen in adult migraine. Sixth, vascular responses to cortical spreading depression are evident in children as shown by perfusion data. Finally, mitochondrial abnormalities have been noted in some migraine patients, but whether all migraine patients are affected by mitochondrial deficiencies is unknown. Low magnesium levels may partially explain mitochondrial disruption. Magnesium as a preventative therapy may be effective for more widespread use, particularly in pediatric populations. All of these findings are based on the sparse literature that investigated pediatric migraine using MRI and spectroscopy and thus should be used as a guide for further research as opposed to providing firm conclusions. The more significant conclusion from this review is the great opportunity for MRI to investigate the underlying nature of migraine, particularly in pediatric populations.
Acknowledgement
The authors thank Lebel Lab for assistance with Figure 1.
Disclosures
F.A. received speaker's honorarium from Allergan and Tribute. F.A. also received consultancy fees from Novartis and Teva. The other authors have no conflicts of interest to declare.
Funding
A.D.H. was supported by the Hotchkiss Brain Institute, Alberta Children’s Hospital Research Institute, and a SickKids Foundation and CIHR-IHDCYH New Investigator Award.
Statement of Authorship
Conception and design of review, acquisition and interpretation of data, and drafting of the review were undertaken by M.E.W. and A.D.H. Revisions were undertaken by M.E.W., F.A., and A.D.H.