Abstract
Alpha-amylase (α-amylase) not long ago has acquire recognition as a possible drug target for the management of diabetes. Here, we have investigated the binding and enzyme activity of α-amylase by hesperidin; a naturally occurring flavanone having wide therapeutic potential. Hesperidin exerted an inhibitory influence on α-amylase activity with an IC50 value of 16.6 µM. Hesperidin shows a significant binding toward α-amylase with a binding constant (Ka) of the order of 104 M−1. The evaluation of thermodynamic parameters (∆H and ∆S) suggested that van der Waals force and hydrogen bonding drive seemingly specific hesperidin-α-amylase complex formation. Glycation and oxidation studies were performed using human serum albumin (HSA) as ideal protein. Hesperidin inhibited fructosamine content ≈40% at 50 µM and inhibited advanced glycation end products (AGEs) formation by 71.2% at the same concentration. Moreover, significant recovery was evident in free –SH groups and carbonyl content of HSA. Additionally, molecular docking also entrenched in vitro observations and provided an insight into the important residues (Trp58, Gln63, His101, Glu233, Asp300, and His305) at the heart of hesperidin-α-amylase interaction. This study delineates mechanistic insight of hesperidin-α-amylase interaction and provides a platform for use of hesperidin to treat AGEs directed diseases.







Similar content being viewed by others
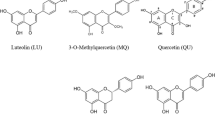
References
Dubey, S., Ganeshpurkar, A., Ganeshpurkar, A., Bansal, D., & Dubey, N. (2017). Glycolytic enzyme inhibitory and antiglycation potential of rutin. Future Journal of Pharmaceutical Sciences, 3(2), 158–162
Sabharwal, R., & Mahajan, A. (2020). Diabetes mellitus, dyslipidemia: Cause for acute myocardial infarction. JK Science, 22(1), 1–2
Asadipooya, K., & Uy, E. M. (2019). Advanced glycation end products (AGEs), receptor for AGEs, diabetes, and bone: Review of the literature. Journal of the Endocrine Society, 3(10), 1799–1818
Younus, H., & Anwar, S. (2016). Prevention of non-enzymatic glycosylation (glycation): Implication in the treatment of diabetic complication. International Journal of Health Sciences, 10(2), 261
Kalousova, M., Skrha, J., & Zima, T. (2002). Advanced glycation end-products and advanced oxidation protein products in patients with diabetes mellitus. Physiological Research, 51(6), 597–604
Adisakwattana, S., Sompong, W., Meeprom, A., Ngamukote, S., & Yibchok-anun, S. (2012). Cinnamic acid and its derivatives inhibit fructose-mediated protein glycation. International Journal of Molecular Sciences, 13(2), 1778–1789
Prasad, C., Davis, K. E., Imrhan, V., Juma, S., & Vijayagopal, P. (2019). Advanced glycation end products and risks for chronic diseases: intervening through lifestyle modification. American Journal of Lifestyle Medicine, 13(4), 384–404
Kotowaroo, M. I., Mahomoodally, M. F., Gurib‐Fakim, A., & Subratty, A. H. (2006). Screening of traditional anti-diabetic medicinal plants of mauritius for possible α‐amylase inhibitory effects in vitro. Phytotherapy Research: An International Journal Devoted to Pharmacological and Toxicological Evaluation of Natural Product Derivatives, 20(3), 228–231
Yao, Y., Sang, W., Zhou, M., & Ren, G. (2010). Antioxidant and α-glucosidase inhibitory activity of colored grains in China. Journal of Agricultural and Food Chemistry, 58(2), 770–774
Kim, Y.-M., Jeong, Y.-K., Wang, M.-H., Lee, W.-Y., & Rhee, H.-I. (2005). Inhibitory effect of pine extract on α-glucosidase activity and postprandial hyperglycemia. Nutrition, 21(6), 756–761
Yousuf, M., Shamsi, A., Khan, P., Shahbaaz, M., AlAjmi, M. F., Hussain, A., & Hassan, M. (2020). Ellagic acid controls cell proliferation and induces apoptosis in breast cancer cells via inhibition of cyclin-dependent kinase 6. International Journal of Molecular Sciences, 21(10), 3526
Yousuf, M., Khan, P., Shamsi, A., Shahbaaz, M., Hasan, G. M., Haque, Q. M. R., Christoffels, A., Islam, A., & Hassan, M. I. (2020). (2020). Inhibiting CDK6 activity by Quercetin is an attractive strategy for cancer therapy. ACS Omega, 5(42), 27480–27491.
Anwar, S., Mohammad, T., Shamsi, A., Queen, A., Parveen, S., Luqman, S., & Asiri, A. M. (2020). Discovery of Hordenine as a potential inhibitor of pyruvate dehydrogenase kinase 3: Implication in lung cancer therapy. Biomedicines, 8(5), 119
Anwar, S., Shamsi, A., Shahbaaz, M., Queen, A., Khan, P., Hasan, G. M., & Ahmad, F. (2020). Rosmarinic acid exhibits anticancer effects via MARK4 inhibition. Scientific Reports, 10(1), 1–13
Akiyama, S., Katsumata, S., Suzuki, K., Nakaya, Y., Ishimi, Y., & Uehara, M. (2009). Hypoglycemic and hypolipidemic effects of hesperidin and cyclodextrin-clathrated hesperetin in Goto-Kakizaki rats with type 2 diabetes. Bioscience, Biotechnology, and Biochemistry, 73(12), 2779–2782
Gandhi, G. R., Vasconcelos, A. B. S., Wu, D.-T., Li, H.-B., Antony, P. J., Li, H., & Gan, R.-Y. (2020). Citrus flavonoids as promising phytochemicals targeting diabetes and related complications: A systematic review of in vitro and in vivo studies. Nutrients, 12(10), 2907
Huang, S., Tsai, S., Lin, J., Wu, C., & Yen, G. (2012). Cytoprotective effects of hesperetin and hesperidin against amyloid β‐induced impairment of glucose transport through downregulation of neuronal autophagy. Molecular Nutrition & Food Research, 56(4), 601–609
Parhiz, H., Roohbakhsh, A., Soltani, F., Rezaee, R., & Iranshahi, M. (2015). Antioxidant and anti‐inflammatory properties of the citrus flavonoids hesperidin and hesperetin: an updated review of their molecular mechanisms and experimental models. Phytotherapy Research, 29(3), 323–331
Roohbakhsh, A., Parhiz, H., Soltani, F., Rezaee, R., & Iranshahi, M. (2015). Molecular mechanisms behind the biological effects of hesperidin and hesperetin for the prevention of cancer and cardiovascular diseases. Life Sciences, 124, 64–74
Zanwar, A. A., Badole, S. L., Shende, P. S., Hegde, M. V., & Bodhankar, S. L. (2014). Cardiovascular effects of hesperidin: A flavanone glycoside. In: Ronald W, Victor P, Sherma Z (Eds.) Polyphenols in human health and disease (pp. 989–992). Elsevier
Rangel-Huerta, O. D., Aguilera, C. M., Martin, M. V., Soto, M. J., Rico, M. C., Vallejo, F., & Mesa, M. D. (2015). Normal or high polyphenol concentration in orange juice affects antioxidant activity, blood pressure, and body weight in obese or overweight adults. The Journal of Nutrition, 145(8), 1808–1816
Peterson, J. J., Beecher, G. R., Bhagwat, S. A., Dwyer, J. T., Gebhardt, S. E., Haytowitz, D. B., & Holden, J. M. (2006). Flavanones in grapefruit, lemons, and limes: A compilation and review of the data from the analytical literature. Journal of Food Composition and Analysis, 19, S74–S80.
Nandakumar, N., & Balasubramanian, M. P. (2012). Hesperidin a citrus bioflavonoid modulates hepatic biotransformation enzymes and enhances intrinsic antioxidants in experimental breast cancer rats challenged with 7, 12-Dimethylbenz (a) anthracene. Journal of Experimental Therapeutics & Oncology, 9, 4
Dhanya, R., & Jayamurthy, P. (2020). In vitro evaluation of anti-diabetic potential of hesperidin and its aglycone hesperetin under oxidative stress in skeletal muscle cell line. Cell Biochemistry and Function, 38(4), 419–427
Li, D., Mitsuhashi, S., & Ubukata, M. (2012). Protective effects of hesperidin derivatives and their stereoisomers against advanced glycation end-products formation. Pharmaceutical Biology, 50(12), 1531–1535
Shamsi, A., Ahmed, A., Khan, M. S., Al Shahwan, M., Husain, F. M., & Bano, B. (2020). Understanding the binding between Rosmarinic acid and serum albumin: In vitro and in silico insight. Journal of Molecular Liquids, 311, 113348
Shamsi, A., Anwar, S., Mohammad, T., Alajmi, M. F., Hussain, A., Rehman, M., & Hassan, M. (2020). MARK4 inhibited by AChE inhibitors, donepezil and rivastigmine tartrate: Insights into Alzheimer’s disease therapy. Biomolecules, 10(5), 789
Shamsi, A., Mohammad, T., Khan, M. S., Shahwan, M., Husain, F. M., Rehman, M., & Islam, A. (2019). Unraveling binding mechanism of Alzheimer’s drug rivastigmine tartrate with human transferrin: Molecular docking and multi-spectroscopic approach towards neurodegenerative diseases. Biomolecules, 9(9), 495
Khan, M.S., Qais, F. A., Rehman, M. T., Ismail, M. H., Alokail, M. S., Altwaijry, N. & & Alqhatani, R. (2020). Mechanistic inhibition of non-enzymaticglycation and aldose reductase activity by naringenin: Binding, enzyme kinetics and molecular docking analysis. International Journal of Biological Macromolecules, 159, 87–97.
Muthenna, P., Suryanarayana, P., Gunda, S. K., Petrash, J. M., & Reddy, G. B. (2009). Inhibition of aldose reductase by dietary antioxidant curcumin: mechanism of inhibition, specificity and significance. FEBS Letters, 583(22), 3637–3642
Ahmed, A., Shamsi, A., Khan, M. S., Husain, F. M., & Bano, B. (2018). Methylglyoxal induced glycation and aggregation of human serum albumin: biochemical and biophysical approach. International Journal of Biological Macromolecules, 113, 269–276
Giannoukakis, N. (2006). Drug evaluation: Ranirestat—an aldose reductase inhibitor for the potential treatment of diabetic complications. Current Opinion in Investigational Drugs (London, England: 2000), 7(10), 916–923
Shamsi, A., Shahwan, M., Husain, F. M., & Khan, M. S. (2019). Characterization of methylglyoxal induced advanced glycation end products and aggregates of human transferrin: Biophysical and microscopic insight. International Journal of Biological Macromolecules, 138, 718–724
Johnson, R. N., Metcalf, P. A., & Baker, J. R. (1983). Fructosamine: A new approach to the estimation of serum glycosylprotein. An index of diabetic control. Clinica Chimica Acta, 127(1), 87–95
Ellman, G. L. (1958). A colorimetric method for determining low concentrations of mercaptans. Archives of Biochemistry and Biophysics, 74(2), 443–450
Zhang, H., Wang, Y., Fei, Z., Wu, L., & Zhou, Q. (2008). Characterization of the interaction between Fe (III)-2, 9, 16, 23-tetracarboxyphthalocyanine and blood proteins. Dyes and Pigments, 78(3), 239–247
Zhang, G., Que, Q., Pan, J., & Guo, J. (2008). Study of the interaction between icariin and human serum albumin by fluorescence spectroscopy. Journal of Molecular Structure, 881(1–3), 132–138
Bolli, A., Marino, M., Rimbach, G., Fanali, G., Fasano, M., & Ascenzi, P. (2010). Flavonoid binding to human serum albumin. Biochemical and Biophysical Research Communications, 398(3), 444–449
Shamsi, A., Mohammad, T., Anwar, S., Alajmi, M. F., Hussain, A., Hassan, M. I., & Islam, A. (2020). Probing the interaction of Rivastigmine Tartrate, an important Alzheimer’s drug, with serum albumin: Attempting treatment of Alzheimer’s disease. International Journal of Biological Macromolecules, 148, 533–542
Rehman, M. T., Shamsi, H., & Khan, A. U. (2014). Insight into the binding mechanism of imipenem to human serum albumin by spectroscopic and computational approaches. Molecular Pharmaceutics, 11(6), 1785–1797
Shi, S., Zhang, Y., Chen, X., & Peng, M. (2011). Investigation of flavonoids bearing different substituents on ring C and their Cu2+ complex binding with bovine serum albumin: structure–affinity relationship aspects. Journal of Agricultural and Food Chemistry, 59(19), 10761–10769
Xiaqing, W. U., Huafang, D., et al. (2018). Exploring inhibitory mechanism of gallocatechin gallate on a-amylase and a-glucosidase relevant to postprandial hyperglycemia. Journal of Functional Foods, 48, 200–209
Lijun, S., Weiqi, C., et al. (2016). Interactions between polyphenols in thinned young apples and porcine pancreatic α-amylase: Inhibition, detailed kinetics and fluorescence quenching. Food Chemistry, 208, 51–60
Alam, M. M., Abul Qais, F., Ahmad, I., Alam, P., Hasan Khan, R., & Naseem, I. (2018). Multi-spectroscopic and molecular modelling approach to investigate the interaction of riboflavin with human serum albumin. Journal of Biomolecular Structure and Dynamics, 36(3), 795–809
Ross, P. D., & Subramanian, S. (1981). Thermodynamics of protein association reactions: Forces contributing to stability. Biochemistry, 20(11), 3096–3102
Zhang, Y., Li, Y., Dong, L., Li, J., He, W., Chen, X., & Hu, Z. (2008). Investigation of the interaction between naringin and human serum albumin. Journal of Molecular Structure, 875(1–3), 1–8
Wang, Y. Q., Zhang, H. M., Zhang, G. C., Tao, W. H., & Tang, S. H. (2007). Interaction of the flavonoid hesperidin with bovine serum albumin: A fluorescence quenching study. Journal of Luminescence, 126(1), 211–218
Tu, B., Wang, Y., Mi, R., Ouyang, Y., & Hu, Y.-J. (2015). Evaluation of the interaction between naringenin and human serum albumin: Insights from fluorescence spectroscopy, electrochemical measurement and molecular docking. Spectrochimica Acta Part A: Molecular and Biomolecular Spectroscopy, 149, 536–543
Förster, T. (1967). Mechanisms of energy transfer. In: MArcel F, Elmer H.S. (Eds.) Comprehensive biochemistry (Vol. 22, pp. 61–80). Elsevier
Jiang, C.-Q., Gao, M.-X., & Meng, X.-Z. (2003). Study of the interaction between daunorubicin and human serum albumin, and the determination of daunorubicin in blood serum samples. Spectrochimica Acta Part A: Molecular and Biomolecular Spectroscopy, 59(7), 1605–1610
Siddiqui, G. A., Siddiqi, M. K., Khan, R. H., & Naeem, A. (2018). Probing the binding of phenolic aldehyde vanillin with bovine serum albumin: Evidence from spectroscopic and docking approach. Spectrochimica Acta Part A: Molecular and Biomolecular Spectroscopy, 203, 40–47
Tundis, R., Loizzo, M. R., & Menichini, F. (2010). Natural products as α-amylase and α-glucosidase inhibitors and their hypoglycaemic potential in the treatment of diabetes: An update. Mini Reviews in Medicinal Chemistry, 10(4), 315–331
Liu, M., Hu, B., Zhang, H., Zhang, Y., Wang, L., Qian, H., & Xiguang, Q. (2017). Inhibition study of red rice polyphenols on pancreatica-amylaseactivity by kinetic analysis and molecular docking. Journal of Cereal Science, 76, 186–192
Sahnoun, M., Trabelsi, S., & Bejar, S. (2017). Citrus flavonoids collectively dominate the α-amylase and α-glucosidase inhibitions. Biologia, 72, 764–773
Zhang, B. W., Li, X., Sun, W. L., Xing, Y., Xiu, Z. L., Zhuang, C. L., & Dong, Y. S. (2017). Dietary flavonoids and acarbose synergistically inhibit α-glucosidase and lower postprandial blood glucose. J Agric Food Chem, 65(38), 8319–8330
Goh, S.-Y., & Cooper, M. E. (2008). The role of advanced glycation end products in progression and complications of diabetes. The Journal of Clinical Endocrinology & Metabolism, 93(4), 1143–1152
Fernandes, A. C. F., Santana, A. L., Martins, I. M., Moreira, D. K. T., Macedo, J. A., & Macedo, G. A. (2020). Anti-glycation effect and the α-amylase, lipase, and α-glycosidase inhibition properties of a polyphenolic fraction derived from citrus wastes. Preparative Biochemistry & Biotechnology, 50(8), 1–9.
Aćimović, J. M., Stanimirović, B. D., & Mandić, L. M. (2009). The role of the thiol group in protein modification with methylglyoxal. Journal of the Serbian Chemical Society, 74(8–9), 867–883
Nita, M., Grzybowski, A. (2016). The role of the reactive oxygen species and oxidative stress in the pathomechanism of the age-related ocular diseases and other pathologies of the anterior and posterior eye segments in adults. Oxidative Medicine and Cellular Longevity, 2016, 23
Beal, M. F. (2002). Oxidatively modified proteins in aging and disease. Free Radical Biology and Medicine, 32(9), 797–803
Balu, M., Sangeetha, P., Murali, G., & Panneerselvam, C. (2005). Age-related oxidative protein damages in central nervous system of rats: modulatory role of grape seed extract. International Journal of Developmental Neuroscience, 23(6), 501–507
Mahmoud, A. M., Mohammed, H. M., Khadrawy, S. M., & Galaly, S. R. (2017). Hesperidin protects against chemically induced hepatocarcinogenesis via modulation of Nrf2/ARE/HO-1, PPARγ and TGF-β1/Smad3 signaling, and amelioration of oxidative stress and inflammation. Chemico-Biological Interactions, 277, 146–158
Bashir, S., Shamsi, A., Ahmad, F., Hassan, M. I., Kamal, M. A. & & Islam, A. (2020). Biophysical elucidation of fibrillation inhibition by sugar osmolytes in α-lactalbumin: multispectroscopic and molecular docking approaches. ACS Omega, 5(41), 26871–26882.
Acknowledgements
The authors extend their appreciation to the Deanship of Scientific Research at the KSU for funding this work through research group project number RGP-215.
Author information
Authors and Affiliations
Corresponding author
Ethics declarations
Conflict of interest
The authors declare no competing interests.
Additional information
Publisher’s note Springer Nature remains neutral with regard to jurisdictional claims in published maps and institutional affiliations.
Supplementary information
Rights and permissions
About this article
Cite this article
Khan, M.S., Rehman, M.T., Ismael, M.A. et al. Bioflavonoid (Hesperidin) Restrains Protein Oxidation and Advanced Glycation End Product Formation by Targeting AGEs and Glycolytic Enzymes. Cell Biochem Biophys 79, 833–844 (2021). https://doi.org/10.1007/s12013-021-00997-8
Received:
Accepted:
Published:
Issue Date:
DOI: https://doi.org/10.1007/s12013-021-00997-8