Abstract
Raw domestic wastewater was used as a culture medium for cellulase production in a bubble column reactor (6.2 UFP/mL, 64.6 U/L h) using the strain Trichoderma harzianum TRIC03-LPBII. Cellulases presented optimum pH and temperature between 4 and 5 and 50 and 70 °C, respectively. Enzymatic extract was concentrated through ultrafiltration and then a cellulolytic formulation was prepared with the addition of sorbitol (50% w/v) and benzoic acid (0.05% w/v). High cellulase stability of around 100% was reached after 30 days at 4 °C. The concentrated extract was also dried in a spray-dryer with the addition of maltodextrin at 20% (w/v), resulting in powder enzymatic formulation with 85% stability after 60 days. With these characteristics, the liquid and powder cellulase products have potential to be used in different industrial applications.







Similar content being viewed by others
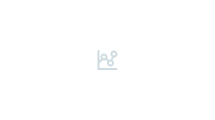
References
Bishof, R. H., Ramoni, J., & Seiboth, B. (2016). Cellulases and beyond: the first 70 years of the enzyme producer Trichoderma reesei. Microbial Cell Factories, 15(1), 1–13.
Alam, M. Z., Muyibi, S. A., & Wahid, R. (2008). Statistical optimization of process conditions for cellulase production by liquid state bioconversion of domestic wastewater sludge. Bioresource Technology, 99(11), 4709–4716.
Libardi, N., Soccol, C. R., Góes-Neto, A., de Oliveira, J., & Vandenberghe, L. P. d. S. (2017). Domestic wastewater as substrate for cellulase production by Trichoderma harzianum. Process Biochemistry, 57, 190–199.
Libardi, N., Soccol, C. R., Carvalho, J. C., & Vandenberghe, L. P. S. (2019). Simultaneous cellulase production using domestic wastewater and bioprocess effluent treatment - a biorefinery approach. Bioresource Technology, 276, 42–50.
Shibuya, H., Magara, K., & Nojiri, M. (2015). Cellulase production by Trichoderma reesei in fed-batch cultivation on soda-anthraquinone pulp of the Japanese cedar. Bulletin of FFPRI, 14(1), 29–35.
Esterbauer, H., Steiner, W., Labudova, I., Hermann, A., & Hayn, M. (1991). Production of Trichoderma cellulase in laboratory and pilot scale. Bioresource Technology, 36(1), 51–65.
Chaplin, M. F., & Bucke, C. (1990). Enzyme Technology. Cambridge: Cambridge University Press.
Himmel, M. E., Abbas, C. A., Baker, J. O., Bayer, E. A., Bomble, Y. J., Brunecky, R., Chen, X., Felby, C., Jeoh, T., Kumar, R., McCleary, B. V., Pletschke, B. I., Tucker, M. P., Wyman, C. E., & Decker, S. R. (2017). Undefined cellulase formulations hinder scientific reproducibility. Biotechnology for Biofuels, 10(283), 1–4.
Singhania, R., Adsul, M., Pandey, A., & Patel, A. (2017). In A. Pandey, S. Negi, & C. Soccol (Eds.), Current Developments in Biotechnology and Bioengineering Production, Isolation and Purification of Industrial Products: Cellulases (pp. 73–97). Amsterdam: Elsevier.
Becker, T., Park, G., & Gaertner, A. L. (1997). In J. H. Van Ee, O. Misset, & E. Baas (Eds.), Enzymes in Detergency: Formulation of Detergent Enzymes (pp. 299–325). New York: Marcel Dekker.
De Paz, R., Barnett, C. C., Dale, D. A., Carpenter, J. F., Gaertner, A. L., & Randolph, T. W. (2000). The excluding effects of sucrose on a protein chemical degradation pathway: methionine oxidation in subtilisin. Archives of Biochemistry Biophysics, 384, 123–132.
Belghith, H., Ellouz Chaabouni, S., & Gargouri, A. (2001). Stabilization of Penicillium occitanis cellulases by spray drying in presence of maltodextrin. Enzyme and Microbial Technology, 28(2–3), 253–258.
Selivanov, A. S. (2005). Stabilization of cellulases using spray drying. Engineering in Life Sciences, 5(1), 78–80.
Ferreira, S., Malacrida, C. R., & Telis, V. R. N. (2016). Influence of emulsification methods and use of colloidal silicon dioxide on the microencapsulation by spray drying of turmeric oleoresin in gelatin-starch matrices. Canadian Journal of Chemical Engineering, 94(11), 2210–2218.
Samantha, S. C., Bruna, A. S. M., Adriana, R. M., Fabio, B., Sandro, A. R., & Aline, R. C. A. (2015). Drying by spray drying in the food industry: micro-encapsulation, process parameters and main carriers used. African Journal of Food Science, 9(9), 462–470.
Anvisa. (1988). Available from: http://portal.anvisa.gov.br/documents/33916/391619/Resolucao_04_1988.pdf/7311a4d9-d5db-44d6-adbd-c7e6891d079d. Acessed January 10, 2018.
ICH. (1995). Available from: https://www.ich.org/products/guidelines/quality/quality-single/article/stability-testing-of-biotechnologicalbiological-products.html. Acessed January 10, 2018.
Mandels, M., & Reese, E. T. (1957). Induction of cellulase in Trichoderma viride as influenced by carbon sources and metals. Journal of bacteriology, 73(2), 269–278.
Camassola, M., & Dillon, A. J. P. (2012). Cellulase determination: modification to make the filter paper assay easy, practical and efficient. Open Access Scientific Reports, 1(125), 2–5.
Miller, G. L. (1959). Use of dinitrosalicylic acid reagent for determination of reducing sugar. Analytical Chemistry, 31(3), 426–428.
Busto, M., & Ortega, N. (1996). Location, kinetics and stability of cellulases induced in Trichoderma reesei cultures. Bioresource Technology, 57(2), 187–192.
Chen, H., Chang, H., Fan, C., Chen, W., Lee, M. (2011). Screening, isolation and characterization of cellulose biotransformation bacteria from specific soils. 2011 International Conference on Environment and Industrial Innovation IPCBEE, 12, 216–220.
Tiwari, P., Misra, B. N., & Sangwan, N. S. (2013). β-Glucosidases from the fungus Trichoderma: an efficient cellulase machinery in biotechnological applications. BioMed Research International, 2013, 1–10.
Sørensen, A., Lübeck, M., Lübeck, P. S., & Ahring, B. K. (2013). Fungal beta-glucosidases: a bottleneck in industrial use of lignocellulosic materials. Biomolecules, 3(3), 612–631.
Yun, S. I., Jeong, C. S., Chung, D. K., & Choi, H. S. (2001). Purification and some properties of a beta-glucosidase from Trichoderma harzianum type C-4. Bioscience, biotechnology, and biochemistry, 65(9), 2028–2032.
Chandra, M., Kalra, A., Sangwan, A., & Sangwan, R. (2013). Biochemical and proteomic characterization of a novel extracellular β-glucosidase from Trichoderma citrinoviride. Molecular Biotechnology, 53(3), 289–299.
Chauve, M., Mathis, H., Huc, D., Casanave, D., Monot, F., Ferreira, N. L., & Lopes Ferreira, N. (2010). Comparative kinetic analysis of two fungal beta-glucosidases. Biotechnology for biofuels, 3(1), 3.
Chen, H., Hayn, M., & Esterbauer, H. (1992). Purification and characterization of two extracellular beta-glucosidases from Trichoderma reesei. Biochimica et Biophysica Acta, 22, 54–60.
Dekker, R. (1986). Kinetic, inhibition, and stability properties of a commercial beta-D-glucosidase (cellobiase) preparation from Aspergillus niger and its suitability in the hydrolysis of lignocellulose. Biotechnology and Bioengineering, 28(9), 1438–1442.
Whitaker, J. R. (1994). Principles of enzymology for the food sciences. New York: CRC Press Book.
Noel, M., & Combes, D. (2003). Rhizomucor miehei lipase: differential scanning calorimetry and pressure/temperature stability studies in presence of soluble additives. Enzyme and Microbial Technology, 33(2-3), 299–308.
Tiwari, A., & Bhat, R. (2006). Stabilization of yeast hexokinase A by polyol osmolytes: correlation with the physicochemical properties of aqueous solutions. Biophysical Chemistry, 124(2), 90–99.
Yadav, J. K., Prakash, V. (2011). Stabilization of α-amylase, the key enzyme in carbohydrates properties alterations at low ph. International Journal of Food Properties, 1182–1196.
Pazhang, M., Mehrnejad, F., Pazhang, Y., Falahati, H., & Chaparzadeh, N. (2016). Effect of sorbitol and glycerol on the stability of trypsin and difference between their stabilization effects in the various solvents. Biotechnology and Applied Biochemistry, 63(2), 206–213.
Lemos, J. L. S., Bon, E. P. S., Santana, M. D. F. E., & Pereira, N. (2000). Thermal stability of xylanases produced by Aspergillus awamori. Brazilian Journal of Microbiology, 31, 206–211.
Amfep. (2018). Available from: amfep.org. Acessed January 2, 2018.
Carneiro, H. C. F., Tonon, R. V., Grosso, C. R. F., & Hubinger, M. D. (2013). Encapsulation efficiency and oxidative stability of flaxseed oil microencapsulated by spray drying using different combinations of wall materials. Journal of Food Engineering, 115(4), 443–451.
Cano-Chauca, M., Stringheta, P. C., Ramos, A. M., & Cal-Vidal, J. (2005). Effect of the carriers on the microstructure of mango powder obtained by spray drying and its functional characterization. Innovative Food Science and Emerging Technologies, 6(4), 420–428.
Chiou, D., & Langrish, T. A. G. (2007). Crystallization of amorphous components in spray-dried powders. Drying Technology, 25(9), 1423–1431.
Funding
This study was financed in part by Coordenação de Aperfeiçoamento de Pessoal de Nível Superior-Brasil (CAPES) (Finance code 001), Conselho Nacional de Desenvolvimento Científico e Tecnológico-Brasil (CNPq), and internal funds of Federal University of Paraná.
Author information
Authors and Affiliations
Corresponding author
Ethics declarations
Conflict of Interest
The authors declare that they have no conflict of interest.
Additional information
Publisher’s Note
Springer Nature remains neutral with regard to jurisdictional claims in published maps and institutional affiliations.
Rights and permissions
About this article
Cite this article
Libardi, N., Soccol, C.R., Tanobe, V.O.A. et al. Definition of Liquid and Powder Cellulase Formulations Using Domestic Wastewater in Bubble Column Reactor. Appl Biochem Biotechnol 190, 113–128 (2020). https://doi.org/10.1007/s12010-019-03075-1
Received:
Accepted:
Published:
Issue Date:
DOI: https://doi.org/10.1007/s12010-019-03075-1