Abstract
Steel-lined pressure tunnels and shafts are constructed to convey water from reservoirs to hydroelectric power plants. They are multilayer structures made of a steel liner, a cracked backfill concrete layer, a cracked or loosened near-field rock zone and a sound far-field rock zone. Designers often assume isotropic behavior of the far-field rock, considering the most unfavorable rock mass elastic modulus measured in situ, and a quasi-static internal water pressure. Such a conventional model is thus axisymmetrical and has an analytical solution for stresses and displacements. However, rock masses often have an anisotropic behavior and such isotropic assumption is usually conservative in terms of quasi-static maximum stresses in the steel liner. In this work, the stresses and displacements in steel-lined pressure tunnels and shafts in anisotropic rock mass are studied by means of the finite element method. A quasi-static internal water pressure is considered. The materials are considered linear elastic, and tied contact is assumed between the layers. The constitutive models used for the rock mass and the cracked layers are presented and the practical ranges of variation of the parameters are discussed. An extensive systematic parametric study is performed and stresses and displacements in the steel liner and in the far-field rock mass are presented. Finally, correction factors are derived to be included in the axisymmetrical solution which allow a rapid estimate of the maximum stresses in the steel liners of pressure tunnels and shafts in anisotropic rock.











Similar content being viewed by others
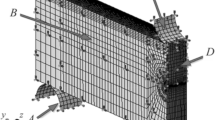
Abbreviations
- E, \(E'\) :
-
Elastic moduli of a transversely isotropic rock
- \(E_c\), \(E_{\rm crm}\), \(E_{\rm rm}\), \(E_s\) :
-
Elastic moduli of the backfill concrete, the near-field rock, the isotropic far-field rock and the steel liner, respectively
- \(E_\theta\) :
-
Elastic modulus in the tangential direction in polar coordinates
- G, \(G'\) :
-
Shear moduli of a transversely isotropic rock
- \(G'_{S-V}\) :
-
Empirical cross-shear modulus of a transversely isotropic rock according to Saint-Venant
- \(G_{\theta r}\), \(G_{\theta z}\) :
-
Shear moduli in polar coordinates
- \(p_c\), \(p_{c, {\rm corr}}\), \(p_{\rm crm}\), \(p_{\rm rm}\) :
-
Pressures transmitted at radii \(r_c\) (and its correction), \(r_{\rm crm}\) and \(r_{\rm rm}\), respectively
- \(p_i\) :
-
Quasi-static internal water pressure
- \(r_c\), \(r_{\rm crm}\), \(r_i\), \(r_{\rm rm}\) :
-
Internal radii of the backfill concrete, the near-field rock, the steel liner and the far-field rock, respectively
- \(t_c\), \(t_{\rm crm}\), \(t_s\) :
-
Thicknesses of the backfill concrete, the near-field rock and the steel liner, respectively
- \(u_r^{c}\), \(u_r^{\rm crm}\), \(u_r^{\rm rm}\), \(u_r^{s}\) :
-
Radial displacements of the backfill concrete, the near-field rock, the far-field rock and the steel liner, respectively
- \(\hat{u}_r^s\), \(\hat{u}_{r,{\rm max}}^s\), \(\hat{u}_{r,{\rm min}}^s\) :
-
Normalized radial displacements in the steel liner, and the maximum and minimum values, respectively
- \(u_{r,{\rm iso}}^s\), \(u_{r,{\rm aniso}}^s\) :
-
Radial displacements in the steel liner considering isotropic and anisotropic rock, respectively
- \(\gamma _{xy}\), \(\gamma _{xz}\), \(\gamma _{yz}\) :
-
Shear strains in Cartesian coordinates
- \(\Delta r_0\) :
-
Initial gap between the steel liner and the backfill concrete
- \(\epsilon _{x}\), \(\epsilon _{y}\), \(\epsilon _{z}\) :
-
Strains in Cartesian coordinates
- \(\theta\) :
-
Angle in polar coordinates
- \(\nu\), \(\nu '\) :
-
Poisson’s ratios of a transversely isotropic rock
- \(\nu _c\), \(\nu _{\rm crm}\), \(\nu _{\rm rm}\), \(\nu _s\) :
-
Poisson’s ratios of the backfill concrete, the near-field rock, the isotropic far-field rock and the steel liner, respectively
- \(\nu _{\theta r}\), \(\nu _{\theta z}\) :
-
Poisson’s ratio in polar coordinates
- \(\sigma _{1}^s\), \(\sigma _{2}^s\), \(\sigma _{3}^s\) :
-
Principal stresses in the steel liner
- \(\sigma _{1,{\rm iso}}^s\), \(\sigma _{1,{\rm aniso}}^s\) :
-
Major principal stresses in the steel liner considering isotropic and anisotropic rocks, respectively
- \(\sigma _{1,{\rm iso}}^{\rm rm}\), \(\sigma _{1,{\rm aniso}}^{\rm rm}\), \(\sigma _{3,{\rm iso}}^{\rm rm}\), \(\sigma _{3,{\rm aniso}}^{\rm rm}\), \(\hat{\sigma }_{1,{\rm iso}}^{\rm rm}\), \(\hat{\sigma }_{1,{\rm aniso}}^{\rm rm}\) :
-
Major and minor principal stresses in the far-field rock considering isotropic and anisotropic rocks, respectively, and their normalized values for the major principal stresses
- \(\hat{\sigma }_{1,{\rm max}}^{s}\), \(\hat{\sigma }_{1}^{s}\) :
-
Normalized maximum and major principal stresses in the steel liner, respectively
- \(\hat{\sigma }_{1}^{\rm rm}\), \(\hat{\sigma }_{1,{\rm max}}^{\rm rm}\), \(\hat{\sigma }_{1,{\rm min}}^{\rm rm}\) :
-
Normalized major principal stresses in the far-field rock, and their maximum and minimum values, respectively
- \(\hat{\sigma }_{3}^{\rm rm}\), \(\hat{\sigma }_{3,{\rm max}}^{\rm rm}\) :
-
Normalized minor principal stresses in the far-field rock and their maximum value, respectively
- \(\hat{\sigma }_{1,{\rm num}}^s\), \(\hat{\sigma }_{{\rm eq,num}}^s\) :
-
Normalized numerical major principal and equivalent stresses in anisotropic rock
- \(\sigma _{1,{\rm corr}}^s\), \(\sigma _{{\rm eq},{\rm corr}}^s\), \(\hat{\sigma }_{1,{\rm corr}}^s\), \(\hat{\sigma }_{{\rm eq},{\rm corr}}^s\), \(\sigma _{1,{\rm corr}}^{\rm rm}\), \(\hat{\sigma }_{1,{\rm corr}}^{\rm rm}\) :
-
Corrected maximum major principal and equivalent stresses in the steel liner and in the far-field rock, and their normalized values, respectively
- \(\sigma _{1,{\rm int}}^s\), \(\sigma _{1,{\rm ext}}^s\) :
-
Major principal stresses at the internal and external fibers, respectively
- \(\sigma _{1,{\rm max}}^s\), \(\sigma _{{\rm eq},{\rm max}}^s\) :
-
Maximum major principal and equivalent stresses in the steel liner
- \(\sigma _{2,{\rm corr}}^s\) :
-
Corrected intermediate principal stress in the steel liner, corresponding to the corrected major principal stress
- \(\sigma _{{\rm eq}}^s\) :
-
Equivalent stress in the steel liner
- \(\sigma _{i}^{s}\), \(\sigma _{i}^{c}\), \(\sigma _{i}^{\rm crm}\), \(\sigma _{i}^{\rm rm}\) :
-
Stresses in the steel liner, the backfill concrete, the near-field rock, the isotropic far-field rock and the steel liner, respectively, along the i-coordinate
- \(\sigma _{x}\), \(\sigma _{y}\), \(\sigma _{z}\) :
-
Stresses in Cartesian coordinates
- \(\tau _{xy}\), \(\tau _{xz}\), \(\tau _{yz}\) :
-
Shear stresses in Cartesian coordinates
- \(X_i\) :
-
Dimensionless parameters
- \(\alpha _i\) :
-
Free coefficients
- FE:
-
Finite element
- FEM:
-
Finite element method
- HSS:
-
High-strength steel
References
Amadei B (1996) Importance of anisotropy when estimating and measuring in situ stresses in rock. Int J Rock Mech Min Sci 33(3):293–325
Amadei B, Savage WZ, Swolfs HS (1987) Gravitational stresses in anisotropic rock masses. Int J Rock Mech Min Sci 24(1):5–14
Amadei B, Swolfs HS, Savage WZ (1988) Gravity-induced stresses in stratified rock masses. Rock Mech Rock Eng 21(1):1–20
ANSYS Inc (2011) ANSYS® Academic research, help system, documentation, release 14.0. ANSYS Inc
ASCE (2012) Steel penstocks, manuals and reports on engineering practice, vol 79. American Society of Civil Engineers, Reston, Virginia
Baslavskii IA (1973) Stresses in the lining of a pressure tunnel driven in an inhomogeneous rock mass. Sov Min 9(6):613–617
Batugin SA, Nirenburg RK (1972) Approximate relation between the elastic constants of anisotropic rocks and the anisotropy parameters. Sov Min 8(1):5–9
Benson R (1989) Design of unlined and lined pressure tunnels. Tunn Undergr Space Technol 4(2):155–170
Bobet A (2009) Elastic solution for deep tunnels. application to excavation damage zone and rockbolt support. Rock Mech Rock Eng 42(2):147–174
Bobet A (2011) Lined circular tunnels in elastic transversely anisotropic rock at depth. Rock Mech Rock Eng 44(2):149–167
Bobet A, Nam SW (2007) Stresses around pressure tunnels with semi-permeable liners. Rock Mech Rock Eng 40(3):287–315
Bowling AJ (2010) Performance of steel liners in the power tunnel of the King River power development, Tasmania. Aust J Civ Eng 6(1):71–80
Brekke TL, Ripley BD (1987) Design guidelines for pressure tunnels and shafts. Technical report, University of California at Berkeley, Department of Civil Engineering, Berkeley, California 94707, EPRI AP-5273, Project 1745–17
Cauvin A, Testa RB (1999) Damage mechanics: basic variables in continuum theories. Int J Sol Struct 36(5):747–761
CECT (1980) Recommendations for the design, manufacture and erection of steel penstocks of welded construction for hydroelectric installations. European Committee of boiler, vessel and pipe work manufacturers
Cerjak H, Enzinger N, Pudar M (2013) Development, experiences and qualifications of steel grades for hydro power conduits. In: Proceedings of the conference on high strength steels for hydropower plants, Graz University of Technology, Graz, Austria
Chène O (2013) In-situ deformation measurement of the Hongrin-Léman shaft. In: Proceedings of the conference on high strength steels for hydropower plants. Graz University of Technology, Graz, Austria
Éristov VS (1967a) Computation of pressure tunnel linings in anisotropic rocks. Hydrotech Constr 1(5):436–442
Éristov VS (1967b) Experimental studies of pressure-tunnel linings in anisotropic formations. Hydrotech Constr 1(12):1054–1057
Gercek H (2007) Poisson’s ratio values for rocks. Int J Rock Mech Min Sci 44(1):1–13
Gerrard CM (1977) Background to mathematical modelling in geomechanics: the roles of fabric and stress history. In: Gudehus G (ed) Finite elements in geomechanics. Wiley, London
Gerrard CM (1982) Equivalent elastic moduli of a rock mass consisting of orthorhombic layers. Int J Rock Mech Min Sci 19:9–14
Gonzaga GG, Leite MH, Corthésy R (2008) Determination of anisotropic deformability parameters from a single standard rock specimen. Int J Rock Mech Min Sci 45:1420–1438
Greiner R, Innerhofer sen G, Stering W (2013) New design aspects for steel linings of pressure shafts made of high strength steel. In: Proceedings of the conference on high strength steels for hydropower plants. Graz University of Technology, Graz, Austria
Hachem FE, Schleiss AJ (2009) The design of steel-lined pressure tunnels and shafts. Int J Hydropower Dams 16(3):142–151
Hachem FE, Schleiss AJ (2011) A review of wave celerity in frictionless and axisymmetrical steel-lined pressure tunnels. J Fluids Struct 27(2):311–328
Hakala M, Kuula H, Hudson J (2007) Estimating the transversely isotropic elastic intact rock properties for in situ stress measurement data reduction: A case study of the Olkiluoto mica gneiss, Finland. Int J Rock Mech Min Sci 44(1):14–46
Hefny AM, Lo KY (1999) Analytical solutions for stresses and displacements around tunnels driven in cross-anisotropic rocks. Int J Numer Anal Methods Geomech 23(2):161–177
Homand F, Morel E, Henry JP, Cuxac P, Hammade E (1993) Characterization of the moduli of elasticity of an anisotropic rock using dynamic and static methods. Int J Rock Mech Min Sci 30(5):527–535
Jing L (2003) A review of techniques, advances and outstanding issues in numerical modelling for rock mechanics and rock engineering. Int J Rock Mech Min Sci 40(3):283–353
Jing L, Hudson JA (2002) Numerical methods in rock mechanics. Int J Rock Mech Min Sci 39(4):409–427
Kumar P, Singh B (1990) Design of reinforced concrete lining in pressure tunnels, considering thermal effects and jointed rockmass. Tunn Undergr Space Technol 5(1/2):91–101
Lekhnitskii SG (1963) Theory of elasticity of an anisotropic elastic body. Holden-Day Inc, San Francisco
Olsson R, Lindblom U, Glamheden R (1997) Design of pressure shafts in recognition of rock structure and stress. Tunnelling Asia ’97. New Delhi, India, pp 615–624
Postol’skaya OK (1986) Effect of the structure of a rock mass and properties of rocks on the stress state of hydraulic pressure tunnels. Hydrotech Constr 20(1):25–30
Ribordy L (1998) Le puits blindé et le répartiteur de l’aménagement Cleuson-Dixence. Wasser, Energie, Luft-Eau, Energie, Air (3/4):53–60
Schleiss AJ (1988) Design criteria applied for the lower pressure tunnel of the North Fork Stanislaus River hydroelectric project in California. Rock Mech Rock Eng 21(3):161–181
Schleiss AJ (2013) Competitive pumped-storage projects with vertical pressure shafts without steel linings / Konkurrenzfähige Pumpspeicherwerkprojekte dank ungepanzerter, vertikaler Druckschächte. Geomech Tunn 6(5):456–463
Schleiss AJ, Manso PA (2012) Design of pressure relief valves for protection of steel-lined pressure shafts and tunnels against buckling during emptying. Rock Mech Rock Eng 45(1):11–20
Seeber G (1985) Power conduits for high-head plants; part two. Water Power Dam Constr 37:95–98
Sharma SC, Sharma MKV, Routela TS (1997) Design of steel liners in pressure shafts for Theri Power Pland (HPP). In: Tunnelling Asia ’97, New Delhi, India
Singh B (1973) Continuum characterization of jointed rock masses: part I—the constitutive equations. Int J Rock Mech Min Sci Geomech Abstr 10(4):311–335
Timoshenko SP, Goodier JN (1970) Theory of elastic stability, 3rd edn. MacGraw-Hill International, Madrid
Tonon F, Amadei B (2003) Stresses in anisotropic rock masses: an engineering perspective building on geological knowledge. Int J Rock Mech Min Sci 40(7–8):1099–1120
Tran Manh H, Sulem J, Subrin D (2014) A closed-form solution for tunnels with arbitrary cross section excavated in elastic anisotropic ground. Rock Mech Rock Eng 48(1):277–288
USACE (1997) Tunnels and shafts in rock (EM 1110-2-2901). US, Army Corps of Engineers
Vigl A (2013) Conventional design of HPP pressure shafts according to G. Seeber, considering the surrounding rock mass. In: Proceedings of the conference on high strength steels for hydropower plants. Graz University of Technology, Graz, Austria
Wittke W (1990) Rock mechanics: theory and applications with Case Histories. Springer, Berlin
Acknowledgments
This study is part of the consortium HydroNet 2: Modern methodologies for design, manufacturing and operation of hydropower plants, a research project funded by the Swiss Competence Center Energy and Mobility (CCEM-CH). The authors acknowledge the contributions to the article by Dr. Pedro Manso from the Swiss Competence Center for Energy Research Supply of Electricity (SCCER-SoE).
Author information
Authors and Affiliations
Corresponding author
Rights and permissions
About this article
Cite this article
Pachoud, A.J., Schleiss, A.J. Stresses and Displacements in Steel-Lined Pressure Tunnels and Shafts in Anisotropic Rock Under Quasi-Static Internal Water Pressure. Rock Mech Rock Eng 49, 1263–1287 (2016). https://doi.org/10.1007/s00603-015-0813-z
Received:
Accepted:
Published:
Issue Date:
DOI: https://doi.org/10.1007/s00603-015-0813-z