Abstract
Previous works on the conversion of sago starch and sago hampas into sago sugar, production of cellobiose from sago fronds, and the current studies on the health benefits from consumption of brown sago sugar are presented in this paper. Hydrolysis of sago starch into sugar generates total (100%) recovery, containing glucose (94%), maltose, and other impurities at 3% each. Purification of the brown sago sugar is achieved using powdered activated charcoal to remove all impurities and color. Drying of the purified and concentrated white sago sugar is best performed in an oven (minimum 60 °C), producing high (100%) yield of sugar crystals after several days. Analysis of sweetness revealed that the sago sugar is as sweet as 50% glucose. Brown sago sugar is preferable to white sago sugar due to the presence of antioxidant, analyzed based on total phenolic content (TPC) at 300 mg/kg sugar. Some residual of the TPC can be detected even after purification of the brown sugar. Sago sugar is also obtainable through enzymatic hydrolysis of physically treated sago hampas, generating substantial amount of sugars (70% w/w). Current research also reveals the feasibility of producing cellobiose (approx. 12% w/w) from fresh sago frond, a type of pharmaceutical sugar which commands a higher price than glucose. It is obvious that sago palm has tremendous potential to be adopted as the new source of sugars to replace cane sugar.
You have full access to this open access chapter, Download chapter PDF
Similar content being viewed by others
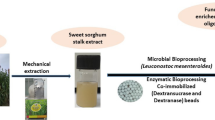
Keywords
22.1 Introduction
In a world starch market dominated by corn, potato, and tapioca, the world production of starch has been estimated to be 27.5 million mt, with an insignificant amount of sago starch consumed, about 3%, 200,000–300,000 mt per annum. Clearly, there is a need to enhance the importance of sago as a major crop in Southeast Asia for global recognition.
The common knowledge about sago is that it thrives in swampy areas or on shallow peat soils without needing copious amounts of pesticides or herbicides (Pei-Lang et al. 2006), quite unlike the cultivation of oil palm. Sago is also a hardy palm, only minimally affected by floods and forest fires. More than 90% of all sago-planting areas in Malaysia are found in Sarawak, East Malaysia. Sago is the world’s highest starch producer per unit area at 25 mt/ha. This is 4 times higher than rice, 5 times wheat, 10 times potato, and almost 17 times that of tapioca (Ishizaki 1997). Since the average annual intake of starch per person is approximately 250 kg, a 1000 ha sago farm could support and save 100,000 people from starvation (Ishizaki 1997).
The most significant agronomic aspect of sago palm is that it multiplies from suckers; hence replanting is not necessary. The author has had the opportunity to visit a sago field owned by a small community of farmers near Pusa in Sarawak, allegedly planted by their great grandparents, over 300 years ago.
Table sugar is derived from sugarcane, a relatively easy and profitable plant to grow but rather ineffective in reproducing naturally (Braun 1999). On a per capita basis, the amount of sugar consumption in Malaysia is about 50 kg (raw equivalent), one of the highest in the region. Cultivation of sugarcane in Malaysia is relatively small, and although the annual production of sugarcane is relatively high at 1.3–1.6 million mt, the sugar recovery is rather low at only 7–10%/kg of fresh weight.
Lack of local raw materials induces heavy dependency of the sugar industry on imported materials (over 90%). Increases in industrial applications of cane sugar naturally lead to a higher price of this commodity (Adam 2010). Hence, a cheaper alternative which can be obtained locally and in abundance is imperative for sugar production.
22.2 Production of Sugars from Sago Starch
Initial studies on the production of sugars were performed in 1 L lab-scale vessels on hydrolysis of various types of starch (sago, corn, tapioca, and sweet potato flour). A modified procedure for enzymatic hydrolysis of sago starch at the optimum parameters is detailed elsewhere (Bujang et al. 2000a).
Typical enzymatic hydrolysis uses Termamyl-12OL (a thermostable α-amylase from Bacillus licheniformis, 120 KNU/g) for liquefaction (0.5 μl/gram of starch) and incubated at 90 °C for 2 h. This is followed by Dextrozyme (a mixture of glucoamylase from Aspergillus niger and pullulanase from 225 AGU/ml) for saccharification (0.6 μl/gram of starch) and incubated at 60 °C for another 4–6 h to produce hydrolyzed sago sugars, or HSS (Bujang et al. 2000b; Bujang and Jobli 2002). This period may be extended for larger volumes of hydrolysis (Bujang and Law 2006). This method generates a 100% recovery of glucose from sago starch.
It was confirmed earlier that 20% (w/v) sago starch is the optimum starch concentration (Bujang et al. 2000a, 2004), at the ideal pH of 6.5 for liquefaction and 4.5 for saccharification, for producing sago sugars at over 100% recovery. The same procedure was performed on other types of starch, and, comparatively, the highest concentration of sugars was obtained from sago starch at 100% recovery, followed by sweet potato (75%), corn (65%), and tapioca starch at 60% (Booty and Bujang 2009).
Increasing the amount of starch from 200 g/L to 10 kg/50 L reduces the glucose recovery by 20%, mostly due to the constraints and capacity of our lab equipment. These results confirmed that it is possible to scale up the process of enzymatic hydrolysis of sago starch with some loss in glucose yield (Booty and Bujang 2009).
22.3 Purification of Sago Sugars
Powdered activated charcoal (PAC) has been used extensively in purification and filtration processes due to its ability to absorb odorous or colored substances from gases or liquids. HSS is centrifuged and filtered to produce brown sago sugar (BSS). Purified sago sugars (PSS), or white sugar, is obtained by PAC filtration of BSS under gravity (2.5 ml/min) which showed a higher (85%) recovery of sugars at a lower amount of PAC (5 g) compared to 10 g PAC (75%). A high recovery of almost 90% was achieved when filtration was enhanced using a pump with a flow rate of 460 ml/h (Bujang et al. 2012).
Ang et al. (2006) reported that adsorption between PAC is lower toward glucose and lactate, but higher toward protein and color. However, the purification process to eliminate other impurities will inadvertently adsorb some sugars, albeit at small concentrations. This was amplified when purification was performed at a higher amount of PAC (10 g) where the yield of sugars recovery was lower.
Purified (white) sago sugar contains mostly glucose (94%), with maltose and other impurities, each at 3%, as shown in Fig. 22.1 (Bujang 2012; Monib 2015). The hydrolytic enzymes used contain α-amylase, which attacks gelatinized starch randomly, thereby producing several types of mono- or oligosaccharides as impurities (3%).
22.4 Drying of Sago Sugars
The crystallization of PSS was studied by several methods, freeze drying, spray drying, and oven drying, resulting in different degrees of success and yield. Spray drying gave the lowest recovery at less than 20%, while both freeze drying and oven drying yielded about 100%—all measured based on weight of the dried sago sugars (DSS) at the end of the process.
Although oven drying (60 °C) clearly requires longer time (3–5 days), this method was adopted for our preliminary and future research at lab-scale processes (Monib 2015). However, the heating process creates a slightly yellow color of the sugar crystals.
22.5 Sweetness Test
Sweetness was measured by making a 10–70% solution of the tested compound in distilled water and then requesting a test panel to taste it. If necessary, the solution was diluted and tasted again until the taster could confirm the sweetness (Yau et al. 1989). The relative sweetness of the various sugars or sweetening agents was compared to sucrose at certain dilutions, compared to the sweetness of sucrose as 100 (Godshall 1996).
The sweetness test was performed by dissolving white crystals of sago sugar in water (20 g/L to 100 g/L) and comparing this to a standard solution of 5% sucrose (Godshall 1996), performed by a panel of 15 volunteers. The panelists were required to taste several times—without actually drinking—the sweetness of the standard solution, which is of known concentration, and compared to the test solution. The panelists were then required to record whether one solution was sweeter than the other, or if they are of the same sweetness, in the appropriate column of the score sheet. The tests concluded that white sago sugar (containing 94% glucose) is 50% less sweet than sucrose, which is the main component in cane sugar (Monib 2015).
22.6 Flavonoids in Brown Sago Sugars
Naturally occurring flavonoids have been known to occur in numerous plants. Flavonoids are classified according to their chemical structure and subdivided into three subgroups: (a) flavones; (b) flavan-3-ols, flavan-4-ols, flavan-3,4-diols, and proanthocyanidins; and (c) anthocyanidins. Flavonoids (e.g., catechins) have been shown to be the most common group of polyphenolic compounds in the human diet and are found universally in foods of plant origin.
Preliminary analyses show the part flavonoids play in affecting the role of allergens, viruses, and carcinogens. In vitro studies reveal that flavonoids also have anti-inflammatory (USFDA 2013a), antimicrobial (USFDA 2013b), and anticancer properties (USFDA 2013c). Chemical analyses have been performed on brown sago sugars in order to determine potential benefits from its consumption.
Antioxidant assay is based on total phenolic content (TPC) and total flavonoid content (TFC). TPC analysis (gallic acid standard) was done on brown and white sago sugars. At 20% (w/v), white sago sugar is still much healthier than cane sugar, while brown sago sugar has the highest total phenolic and total flavonoid contents as shown in Table 22.1 (Bujang et al. 2015). The types of flavonoids identified so far in brown sago sugar are gallic acid, quercetin, and kaempferol. Only the latter remains after purification into white sugar. Obviously, the purification process using PAC removed most of the antioxidant properties.
22.7 Production of Sugars from Sago Hampas
Sago hampas is composed of the solids (fibers and vascular bundles) separated by filtration from sago effluent, also a highly potential source of raw material to produce sugar generated by the sago industry (Fig. 22.2a,b). It has been shown that for 1 mt of dried sago starch produced, at least 1 mt of dried hampas is discharged into the river (Bujang et al. 1996).
It was reported earlier that in some older sago mills, sago hampas can harbor as much as 60–70% starch, trapped within its fibers (Abd-Aziz 2002). Fresh hampas also has high moisture content (70–80%) and needs to be dried if it is to be transported to a processing center. In modern factories, with efficient extraction facilities, waste sago hampas contains less starch (30–45%), as shown in Table 22.2 (Adeni et al. 2009).
Sago hampas has been treated using steam and hydrolyzed using cellulase, β-glucosidase, and an enzyme complex at the optimum concentration of 20 and 1.5% (v/w), respectively. The optimum digestion period was 20 h for cellulase and 16 for β-glucosidase. At the maximum substrate concentration of 6% (w/v), enzymatic hydrolysis under these optimum conditions generated 30 g/L of sugars or a recovery of 50% from dried sago hampas (Janggu and Bujang 2009).
Adeni et al. (2013) reported that recycling the hydrolysate in enzymatic hydrolysis of sago hampas for production of sugar greatly increased the yield from one cycle (27.8 g/L) to two cycles (73.0 g/L) and three cycles (138.5 g/L), a recovery of 70% from sago hampas (w/w). Fermentation of this sugar using baker’s yeast generated 40.3 g/L of ethanol after 16 h or 93.3% theoretical yield based on glucose concentration.
22.8 Cellobiose from Sago Leaves
Apart from sago starch, a current study is looking at the possibility of producing cellobiose, a type of non-table sugar from sago leaves. Cellobiose can be obtained from enzymatic hydrolysis of cellulosic by-products, in this case sago leaves. The hard epidermis from fresh sago leaves is removed, and then the leaves chopped into 2 cm cubes, pulverized, and boiled for 30 min. The mixture is filtered and the tender solid hydrolyzed using a cellulase enzyme complex (containing cellobiohydrolases, endoglucanases, and β-glucosidases). Preliminary results showed the yield of cellobiose and glucose from fresh sago at 12 and 5%, respectively (Ahmad 2015). Cellobiose is a disaccharide, formed from 2 glucose molecules. Although it is not appropriate table sugar, and generally not consumed, it has several prebiotic properties especially in fermented dairy products, including bifidobacteria. Due to this, the market price for refined cellobiose (about USD 2000/kg) is much higher than glucose.
22.9 Conclusion
The main and only disadvantage of the sago crop is that the initial waiting period for harvest is 8–10 years, which could be one of the reasons for hectares of sago land being cleared for oil palm farming. The main benefit of producing cellobiose is that sago farmers would no longer have to wait so long to receive income from the first cycle of sago harvest, since pruned leaves and suckers have been shown to be potential raw materials to yield cellobiose. Sarawak exported 63,000 mt of food-grade sago starch in 2014, with a total value among the different starch types and prices at USD 29.3 million.
Locally, sago starch is sold at USD 500/mt ex-factory price. Converting this even into unrefined sugar can be profitable when compared to cane sugar at USD 800/mt. Bioconversion of sago starch into pure glucose would be much more lucrative since it fetches a higher price to sago starch at USD 12,600/mt. The overall cost for such enzymatic hydrolysis (excluding electricity and labor) has been approximated to be about USD 10/mt of starch, with a calculated profit of about USD 12000/mt.
References
Abd-Aziz S (2002) Sago starch and its utilization: a review. J Biosci Bioeng 94(6):526–529
Adam (2010) Parliament: Malaysia imports 99% sugar supply. Kuala Lumpur, Kuala Lumpur
Adeni DSA, Janggu U, Abd-Aziz S et al (2009) Glucose recovery from sago hampas for ethanol fermentation. In: Proceedings 1st ASEAN sago symposium. Kuching, Sarawak, Malaysia, pp 54–57
Adeni DSA, Bujang KB, Hassan MA, Abd-Aziz S (2013) Recovery of glucose from residual starch of sago hampas for bioethanol production. Biomed Res Int 2013:935852
Ahmad MN (2015) Production of cellobiose from sago frond as antimicrobial agent. Progress report MSc Project. Department of Molecular Biology. Faculty of Resource Science and Technology. Universiti Malaysia Sarawak. Kota Samarahan, Sarawak, Malaysia
Ang SY, Bujang KB, Adeni DSA (2006) Purification of lactic acid from hydrolysed sago starch fermentation by powdered activated carbon (PAC): Adsorption isotherm and kinetic Studies. In: Proceedings 31st Annual conference Malaysian Society Biochem & Mol Biol, 17th August 2006
Booty HB, Bujang KB (2009) Maximising production of sugars from enzymatic hydrolysis of various starch sources, compared to sago starch. In: Proceedings of the 1st ASEAN sago symposium. Kuching. 29–30 October 2009, pp 70–73
Braun T (1999) Sugarcane. Retrieved October 1, 2010, from Ethnobotanical Leaflets. http://www.ethnoleaflets.com
Bujang KB (2012) A holistic approach to maximise productivities of the sago industry. Plenary speaker. In: Proceedings 2nd ASEAN sago symposium. Co-organised by UNIMAS, CRAUN, IPB & FAO. 29–30 October 2012, pp 25–27
Bujang KB, Jobli S (2002) Effects of glucose feed concentrations on continuous lactate production from sago starch. In: International symposium tropical natural bio-resources and green chemistry strategy. ICBiotech (Osaka, Japan). October 27–30, 2002, pp 358–363
Bujang KB, Law PL (2006) Pilot-scale production of sugars from sago starch. Bio-Malaysia 2006 Kuala Lumpur Convention Centre 6–8 December 2006
Bujang KB, Apun K, Salleh MA (1996) A study in the production and bioconversion of sago waste. In: Proceedings 6th international sago symposium. Sago: the future source of food and feed. Universitas Riau, Pekanbaru, Sumatra. 9–12 December 1996, pp 193–200
Bujang KB, Adeni DSA, Jolhiri P (2000a) Effects of starch concentration and pH on enzymatic hydrolysis of sago starch. In: Biotechnology for sustainable utilisation of biological resources in the tropics, vol 14. ICBiotech, Osaka, pp 32–35
Bujang KB, Jolhiri P, Ishizaki A (2000b) Effects of pH on production of lactate from hydrolysed sago starch utilising Lactococcus lactis IO-1. ICBiotech (Osaka Japan) 14:27–31
Bujang KB, Bujang S, Adeni DSA (2004) Effects of calcium carbonate in fermentation of L-lactic acid from hydrolyzed sago starch, vol 26. ICBiotech, Osaka, pp 637–643
Bujang KB, Monib NJ, Nolasco-Hipolito C (2012) Production and purification of sago sugar. In: Proceedings 2nd ASEAN sago symposium. Coorganised by UNIMAS, CRAUN, IPB & FAO. 29–30 October 2012, pp 171–176
Bujang KB, Hussaini AASA, Adeni DSA et al (2015) Total phenolic, flavonoid content, and antioxidant activity of sago sugar. R&D Expo 2015. Organised by RIMC. Universiti Malaysia Sarawak, Kota Samarahan, Sarawak, Malaysia
Godshall MA (1996) Flavour components of cane sugars. Sugar Processing Research Institute, Inc, New Orleans
Ishizaki A (1997) Concluding remarks for the 6th international sago symposium. Riau Indonesia Sago Comm 8(2):22–24
Janggu U, Bujang KB (2009) Maximizing sugar production from enzymatic hydrolysis of sago fiber for ethanol fermentation. In: Proceedings 1st ASEAN sago symposium. Kuching, Sarawak, Malaysia. 29–30 October 2009, pp 30–33
Monib N J (2015) Development on sago sugars recovery and purification by filtration on powdered activated carbon (PAC). Master’s Thesis. Dept. of Molecular Biology. Faculty of Resource Science and Technology. Universiti Malaysia Sarawak. Kota Samarahan, Sarawak, Malaysia
Pei-Lang AT, Mohamed AMD, Karim AA (2006) Sago starch and composition of associated components in palms of different growth stages. Carbohydr Polym 63:283–286
USFDA (2013a) FDA approved drug products. US Food and Drug Administration. Retrieved 8 November 2013
USFDA (2013b) Health claims meeting significant scientific agreement. US Food and Drug Administration. Retrieved 8 November 2013
USFDA (2013c) Inspections, compliance, enforcement, and criminal investigations (flavonoid sciences). US Food and Drug Administration. Retrieved 8 November 2013
Yau NJN, McDaniel MR, Bodyfelt FW (1989) Sensory evaluation of sweetened flavoured carbonated milk beverages. J Dairy Sci 72:367–377
Acknowledgments
The author wishes to acknowledge assistance from the Sago Chair of UNIMAS, NEDO Grant and JSPS Programs from Japan, and IRPA and MOSTI grants from Malaysia, together with cooperation from the State Government of Sarawak and the Sarawak Sago Association, for their financial and logistics support of these studies over the past 15 years.
Author information
Authors and Affiliations
Corresponding author
Editor information
Editors and Affiliations
Rights and permissions
This chapter is published under an open access license. Please check the 'Copyright Information' section either on this page or in the PDF for details of this license and what re-use is permitted. If your intended use exceeds what is permitted by the license or if you are unable to locate the licence and re-use information, please contact the Rights and Permissions team.
Copyright information
© 2018 The Author(s)
About this chapter
Cite this chapter
Bujang, K. (2018). Production, Purification, and Health Benefits of Sago Sugar. In: Ehara, H., Toyoda, Y., Johnson, D. (eds) Sago Palm. Springer, Singapore. https://doi.org/10.1007/978-981-10-5269-9_22
Download citation
DOI: https://doi.org/10.1007/978-981-10-5269-9_22
Published:
Publisher Name: Springer, Singapore
Print ISBN: 978-981-10-5268-2
Online ISBN: 978-981-10-5269-9
eBook Packages: Biomedical and Life SciencesBiomedical and Life Sciences (R0)