Abstract
In order to investigate rice functional centromeres, OsCENH3-GFP chimeric gene was constructed and transformed into the indica rice variety, Zhongxian 3037, mediated by Agrobacturium. The integration of the exogenous genes in the transgenic plants was confirmed by PCR and Southern blotting. The transgenic plants grow normally during their whole life time, just like Zhongxian 3037. No significant defects were detected in either mitosis or meiosis of the transgenic plants. The overlapping of GFP signals and anti-CENH3 foci in both mitotic and meiotic cells from T0 and T1 generation plants indicated that GFP had been successfully fused with CENH3, so the GFP signals can well represent the CENH3 locations on each chromosome. To evaluate the applicability of the transgenic plants to other genetic studies, fluorescence in situ hybridization (FISH) using rice centromeric tandem repetitive sequence CentO as the probe was conducted on the zygotene chromosomes of pollen mother cells (PMCs). It has been revealed that the GFP signals are overlapping with CentO FISH signals, showing that CentO is one of the key elements constituting rice functional centromeres. Immunofluorescent staining using anti-α-tublin antibody and anti-PAIR2 antibody on the chromosomes during mitosis and meiosis stages of the transgenic plants further reveals that OsCENH3-GFP transgenic plants can be widely used for studying rice molecular biology, especially for tagging functional centromeres in both living cells and tissues.
Similar content being viewed by others
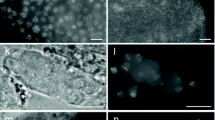
References
Cheeseman I M, Drubin D G, Barnes G. Simple centromere, complex kinetochore: Linking spindle microtubules and centromeric DNA in budding yeast. J Cell Biol, 2002, 157: 199–203
Schueler M G, Higgins A W, Rudd M K, et al. Genomic and genetic definition of a functional human centromere. Science, 2001, 294: 109–115
Rudd M K, Schueler M G, Willard H F. Sequence organization and functional annotation of human centromeres. Cold Spring Harb Symp Quant Biol, 2003, 68: 141–149
Wevrick R, Willard H F. Long-range organization of tandem arrays of α satellite DNA at the centromeres of human chromosomes: High frequency array-length polymorphism andmeiotic stability. Proc Natl Acad Sci USA, 1989, 86: 9394–9398
Kuznetsova I, Podgornaya O, Ferguson-Smith M A. High-resolution organization of mouse centromeric and pericentromeric DNA. Cytogenet Genome Res, 2006, 112: 248–255
Kumekawa N, Hosouchi T, Tsuruoka H, et al. The size and sequence organization of the centromeric region of Arabidopsis thaliana chromosome 5. DNA Res, 2000, 7: 315–321
Kumekawa N, Hosouchi T, Tsuruoka H, et al. The size and sequence organization of the centromeric region of Arabidopsis thaliana chromosome 4. DNA Res, 2001, 8: 285–290
Hosouchi T, Kumekawa N, Tsuruoka H, et al. Physical map-based sizes of the centromeric regions of Arabidopsis thaliana chromosomes 1, 2, and 3. DNA Res, 2002, 9: 117–121
Neumann P, Yan H H, Jiang J M. The centromeric retrotransposons of rice are transcribed and differentially processed by RNAi. Genetics, 2007, 176: 749–761
Ma J, Jackson S A. Retrotransposon accumulation and satellite amplification mediated by segmental duplication facilitate centromere expansion in rice. Genome Res, 2006, 16: 251–259
Yan H H, Ito H, Nobuta K, et al. Genomic and genetic characterization of rice Cen3 reveals extensive transcription and evolutionary implications of a complex centromere. Plant Cell, 2006,18: 2123–2133
Zhang W L, Yi C D, Bao W D, et al. The transcribed 165-bp CentO satellite is the major functional centromeric element in the wild rice species Oryza punctata. Plant Physiol, 2005, 139: 306–315
Nagaki K, Neumann P, Zhang D, et al. Structure, divergence, and distribution of the CRR centromeric retrotransposon family in rice. Mol Biol Evol, 2005, 22:845–855
Cheng Z K, Dong F G, Langdon T, et al. Functional rice centromeres are marked by a satellite repeat and a centromere-specific retrotransposon. Plant Cell, 2002, 14: 1691–1704
Dong F G, Miller J T, Jackson S A, et al. Rice (Oryza sativa) centromeric regions consist of complex DNA. Proc Natl Acad Sci USA, 1998, 95: 8135–8140
Vaughan D A, Morishima H, Kadowaki K. Diversity in the Oryza genus. Curr Opin Plant Biol, 2003, 6: 139–146
Bao W D, Zhang W L, Yang Q Y, et al. Diversity of centromeric repeats in two closely related wild rice species, O. officinalis and O. rhizomatis. Mol Genet Genomics, 2006, 275: 421–430
Amor D J, Kalitsis P, Sumer H, et al. Building the centromere: From foundation proteins to 3D organization. Trends Cell Biol, 2004, 14: 359–368
Palmer D K, O’Day K, Wener M H, et al. A 17-kD centromere protein (CENP-A) copurifies with nucleosome core particles and with histones. J Cell Biol, 1987, 104: 805–815
Palmer D K, O’Day K, Trong H L, et al. Purification of the centromere-specific protein CENP-A and demonstration that it is a distinctive histone. Proc Natl Acad Sci USA, 1991, 88: 3734–3738
Jiang J M, Birchler J A, Parrott W A, et al. A molecular view of plant centromeres. Trends Plant Sci, 2003, 8: 570–575
Talbert P B, Masuelli R, Tyagi A P, et al. Centromeric localization and adaptive evolution of an Arabidopsis histone H3 variant. Plant Cell, 2002, 14: 1053–1066
Zhong C X, Marshall J B, Topp C, et al. Centromeric retroelements and satellites interact with maize kinetochore protein CENH3. Plant Cell, 2002, 14: 2825–2836
Nagaki K, Cheng Z K, Ouyang S, et al. Sequencing of a rice centromere uncovers active genes. Nat Genet, 2004, 36: 138–145
Malik H S, Henikoff S. Adaptive evolution of Cid, a centromerespecific histone in Drosophila. Genetics, 2001, 157: 1293–1298
Warburton P E, Cooke C A, Bourassa S, et al. Immunolocalization of CENP-A suggests a distinct nucleosome structure at the inner kinetochore plate of active centromeres. Curr Biol, 1997, 7: 901–904
Yoda K, Ando S, Morishita S, et al. Human centromere protein A (CENP-A) can replace histone H3 in nucleosome reconstitution in vitro. Proc Natl Acad Sci USA, 2000, 97: 7266–7271
Westermann S, Cheeseman I M, Anderson S, et al. Architecture of the budding yeast kinetochore reveals a conserved molecular core. J Cell Biol, 2003, 163: 215–222
Blower M D, Karpen G H. The role of Drosophila CID in kinetochore formation, cell-cycle progression and heterochromatin interactions. Nat Cell Biol, 2001, 3: 730–739
Howman E V, Fowler K J, Newson A J, et al. Early disruption of centromeric chromatin organization in centromere protein A (Cenpa) null mice. Proc Natl Acad Sci USA, 2000, 97: 1148–1153
Blower M D, Sullivan B A, Karpen G H. Conserved organization of centromeric chromatin in flies and humans. Dev Cell, 2002, 2: 319–330
Niwa Y, Hirano T, Yoshimoto K, et al. Non-invasive quantitative detection and applications of non-toxic, S65T-type green fluorescent protein in living plants. Plant J, 1999, 18: 455–463
Yu H X, Liu Q Q, Wang L, et al. Optimization of Agrobacterium-mediated transformation of rice mature embryos and regeneration of transgenic plants with metr gene (in Chinese). Hereditas, 2005, 27: 756–762
Murray M G, Thompson W F. Rapid isolation of high molecular weight plant DNA. Nucleic Acid Res, 1980, 8: 4321–4325
Sambrook J, Fritsch E F, Maniatis T. Molecular Cloning: A Laboratory Manual. 2nd ed. Cold Spring Harbor: Cold Spring Harbor Laboratory Press, 1989
Zhang D, Yang Q, Bao W, et al. Molecular cytogenetic characterization of the Antirrhinum majus genome. Genetics, 2005, 169: 325–335
Nonomura K I, Nakano M, Eiguchi M, et al. PAIR2 is essential for homologous chromosome synapsis in rice meiosis I. J Cell Sci, 2006, 119: 217–225
Malik H S, Henikoff S. Phylogenomics of the nucleosome. Nat Struct Biol, 2003, 10: 882–891
Lermontova I, Schubert V, Fuchs J, et al. Loading of Arabidopsis centromeric histone CENH3 occurs mainly during G2 and requires the presence of the histone fold domain. Plant Cell, 2006, 18: 2443–2451
Author information
Authors and Affiliations
Corresponding author
Additional information
Contributed equally to this work
Supported by the National Natural Science Foundation of China (Grants Nos. 30770131, 30530070 and 30671285) and High Technology Research and Development Program of China (Grant No. 2006AA02Z124)
About this article
Cite this article
Yu, H., Wang, X., Gong, Z. et al. Generating of rice OsCENH3-GFP transgenic plants and their genetic applications. Chin. Sci. Bull. 53, 2981–2988 (2008). https://doi.org/10.1007/s11434-008-0376-4
Received:
Accepted:
Published:
Issue Date:
DOI: https://doi.org/10.1007/s11434-008-0376-4